Thermal stability and spectroscopic study of Ho3+/Yb3+ co-doped fluorophosphates glasses
⁎Corresponding author. bentouila.om@univ-ouargla.dz (O. Bentouila)
-
Received: ,
Accepted: ,
This article was originally published by Elsevier and was migrated to Scientific Scholar after the change of Publisher.
Peer review under responsibility of King Saud University.
Abstract
Holmium/Ytterbium co-doped fluorophosphate glasses with compositions (80-x-y) NaPO3-10SrF2-10ZnF2- xHoF3- yYbF3 (x = 1 and y = 0.5, 1, 1.5 and 2 mol%) were prepared by melt-quenching technique. The stability criteria indicate that these glasses exhibit a good resistance against devitrification. Densities of glasses were determined and showed an almost linear variation with increase of YbF3 content. Spectroscopic parameters of Ho3+ such as radiative transition probability, branching ratio, spectroscopic quality factor, integrated emission cross section and radiative lifetime, were calculated on the basis of Judd-Ofelt analysis. The results showed that these glasses could be proposed as suitable lasing materials.
Keywords
Holmium
Ytterbium
Rare earth
Fluorophosphates glass
Judd-Ofelt theory
1 Introduction
Rare earth ions doped glasses have a potential application in different fields as a solid state lasers, optical fiber amplifiers and waveguide lasers. Rare earth ions are used as doping agents because of their various transitions in the visible and infrared regions and their insensitivity to the matrix in which are introduced. Up to now, a variety of glassy materials such as silicate (Bai et al., 2011), phosphate (Rivera-López et al., 2011; Hraiech et al., 2013), fluoride (Mortier et al., 2007; Reichert et al., 2015), chalcogenide (Li et al., 2016; Ari et al., 2017), tellurite (Meruva et al., 2014; Costa et al., 2015; Sajna et al., 2016), germanate (Fan et al., 2011, 2015) and halogeno-phosphates glasses (Babu and Ratnakaram, 2016; Galleani et al., 2017) have been investigated as host materials for rare earth ions in the aim to developing of optical devices. Among different glassy host materials, halogeno-phosphates glasses offer significant advantages. They are easily prepared by introducing selected metal halides (fluoride in our case) into polyphosphate glasses. These glasses exhibit low phonon energy than that of phosphate glasses and a less complex fabrication route than that of fluoride glasses and can accept a high concentration of rare earth ions which can improve or induce new optical properties (Poulain et al., 1992; Kenyon, 2002; Dwivedi et al., 2010; Polishchuk et al., 2011; Galleani et al., 2017).
Solid state lasers operating at 2 μm wavelength has attracted so much attention in recent years owing to their potential applications in several fields such as eye safe laser radars, remote sensing, military, atmospheric pollution detection, medical surgery, etc (Tian et al., 2010; Wu et al., 2012; Ryabochkina et al., 2017). Efficient 2 μm emission required an appropriate rare earth ions and host materials. 2 µm laser emission can be achieved in some rare earth ions such as Ho3+ with the transition 5I7 → 5I8 (Fan et al., 2015) However, Ho3+ ions have no corresponding absorption energy levels to be pumped directly by the commercial 800 nm or 980 nm laser diode (LD). Thus, Co-doping with other rare earth ions such as Tm3+ (Seshadri et al., 2014; Chen et al., 2016) and Yb3+ (Żmojda et al., 2012; Pandey et al., 2016) ions can sensitize efficiently Ho3+ to achieve 2 µm emission. Yb3+ is frequently chosen as a sensitizer because this ion has a strong absorption band around 980 nm, and the Yb3+: 2F5/2 level is close to the Ho3+: 5I6 level which can provide an efficient energy transfer from Yb3+ to Ho3+ ions.
A several number of recent studies reporting the spectroscopic properties of Ho3+ doped glasses and Ho3+ doped glasses sensitized with either Tm3+,Yb3+ or Er3+ are available in the literature (Babu and Ratnakaram, 2016; Bai et al., 2011; Chen et al., 2016; Gandhi et al., 2010; Hemming et al., 2014; Rai et al., 2003; Satyanarayana et al., 2010). Furthermore, to our knowledge, there are few number of investigations on Ho3+/Yb3+ co-doped fluorophosphate glasses (Gámez et al., 2009; Tian et al., 2010; Wang et al., 2011). In this work we report thermal and spectroscopic studies of a new composition of fluorophosphate glasses co-doped Ho3+/Yb3+ with doping concentration of Yb3+ varied from 0.5 mol% up to 2 mol% while the doping concentration of Ho3+ is kept constant at 1 mol%.
2 Experimental
2.1 Glass preparation
Samples of fluorophosphate glasses with general formula (in mol%): (80-x-y) NaPO3-10SrF2-10ZnF2- xHoF3- yYbF3 (x = 1 and y = 0.5, 1, 1.5 and 2 mol%) were prepared using high-purity of NaPO3, SrF2, ZnF2, HoF3 and YbF3 powder. The doping concentration of the rare earth was set at 1 mol% for Ho3+ ions and varying from 0.5 to 2 mol% for Yb3+ ions. A stoichiometric mixture of the starting materials (about 10 g) is placed in a platinum crucible and melted at 900 °C for 15 min. The melts were cast on preheated brass molds at a temperature 10 °C below the glass transition temperature. Thermal annealing of the samples is performed for several hours, in order to eliminate the internal stresses of mechanical or thermal origins created during quenching, following by a slow cooling to room temperature. After annealing, the glass samples were cut and polished. The samples obtained after polishing have parallel faces allowing the optical characterization. In the other hand, some local defects, such as scratches and unevenness, are difficult to avoid.
2.2 Measurements
The characteristic temperatures (temperature of glass transition Tg and temperature of onset crystallization Tx) were determined by differential scanning calorimetry (DSC) using DSC TA Instrument with a programmed heating rate of 10 °C/min. The accuracy on the temperature is about ±2 °C. Density was measured by Archimedean method with an accuracy of ±0.001. Refractive index was measured by an Abbe refractometer with a mean error ±0.001. Absorption spectra were recorded at room temperature using a double beam spectrophotometer UV–Vis – Near IR CARY 5G brand operating between 200 and 3000 nm with a spectral resolution of 0.1 nm.
3 Results
3.1 Glass samples
Fig. 1 shows all the samples: non-doped, doped with Ho3+ only and co-doped H3+/Yb3+. Compositions of glass samples were presented in Table 1.

- Fluorophosphates glass Samples.
Name of the sample | Glass compositions (mol%) | ||||
---|---|---|---|---|---|
NaPO3 | SrF2 | ZnF2 | HoF3 | YbF3 | |
NPZS | 80 | 10 | 10 | 0 | 0 |
NPZS_H1 | 79 | 10 | 10 | 1 | 0 |
NPZS_HY0.5 | 79.5 | 10 | 10 | 1 | 0.5 |
NPZS_HY1 | 78 | 10 | 10 | 1 | 1 |
NPZS_HY1.5 | 77.5 | 10 | 10 | 1 | 1.5 |
NPZS_HY2 | 77 | 10 | 10 | 1 | 2 |
3.2 Thermal properties, density and refractive index
Fig. 2 represents the DSC curves of the samples:j without any doping ions (dash-dotted line), doped with Ho3+ only (dotted line) and Ho3+/Yb3+ co-doped (with 1 mol%YbF3, solid line). Glass transition temperature Tg for NPSZ_HYy series still situated around 250 °C and the crystallization onset Tx around 450 °C.
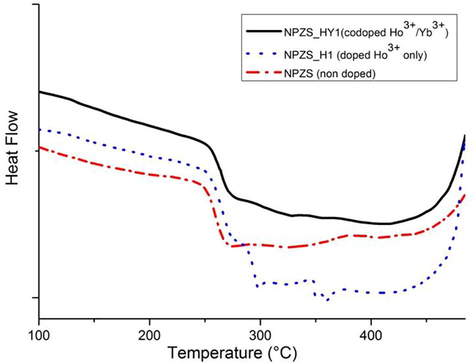
- DSC Curves of the non-doped, Ho3+ doped and Ho3+/Yb3+ co-doped glass samples.
The values of refractive index and the density of glass samples were presented in Table 2. The results show a small almost linear variation of refractive index and density with the variation of YbF3 content.
Samples | Refractive index ±0.001 |
Density (g/cm3) ±0.001 |
---|---|---|
NPZS | 1.494 | 2.772 |
NPZS_H1 | 1.495 | 2.803 |
NPZS_HY0.5 | 1.493 | 2.820 |
NPZS_HY1 | 1.494 | 2.859 |
NPZS_HY1.5 | 1.495 | 2.883 |
NPZS_HY2 | 1.496 | 2.904 |
3.3 Absorption spectra and Judd-Ofelt analysis
The absorption spectra are shown in Fig. 3. We can count ten absorption bands centered at 1957, 1155, 642, 538, 486, 472, 448, 418, 386 and 360 nm corresponding to the optical transitions of the Ho3+ ion from its ground state 5I8 to the different excited states: 5I7, 5I6, 5F5, 5F4, 5F3, (5F2, 3K8), 5G6, 5G5, 5G4 and 3H6 respectively. We can also observe an absorption band at 980 nm correspond to the transition 2F7/2 to 2F5/2 of the Yb3+ ion in the four co-doped samples.
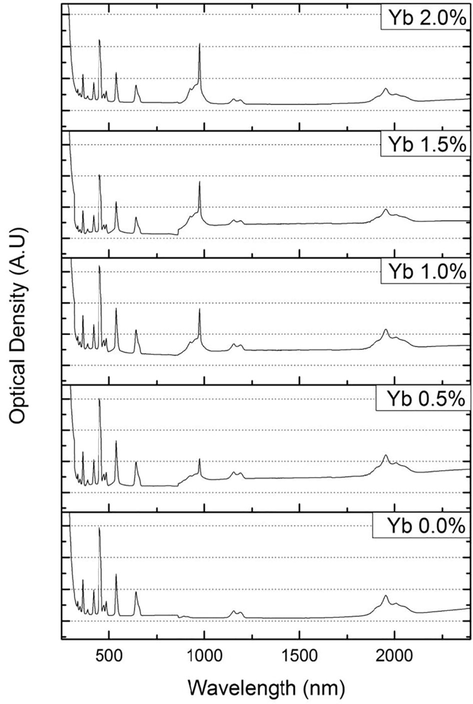
- Absorption spectra of NPZS_HY samples.
The different spectroscopic parameters of the trivalent rare-earth ions in various hosts can be calculated by the application of the theory proposed by Judd (Judd, 1962) and Ofelt (Ofelt, 1962). The detailed assumptions of the theory have been described in original articles. A brief summary of the theory will present below taking into account only the formulas necessary to determine the different spectroscopic parameters.
The intensities of intraconfigurational f-f transitions of trivalent rare earths observed in the absorption spectra can be described by the oscillator strengths fmes of each J → J′ transition. The majority of these transitions are induced electric dipole transitions, although a few magnetic dipole transitions are also present. From the absorption spectra, we can calculate the oscillator strength fmes, which is proportional to the band absorption intensity, from the value of the absorption coefficient α(λ) at a particular wavelength λ according to the formula:
On the other side, the oscillator strength can be given in term of the electric dipole line strength Sed:
The values of the Ωk are empirically determined by comparing the computed values starting from the formula (2) with the values obtained from the absorption spectra at ambient temperature of the oscillator strengths (formula (1)). If q is the number of the absorption bands considered in experiments, the resolution of a system of q equation to 3 unknown factors, by a least square approximation, makes it possible to obtain the values of the parameters Ωk. These parameters are expressed in cm2. A measure of the accuracy of the fit is given by the root mean square deviation (RMS):
The values of Judd-Ofelt parameters obtained are used to calculate the line strength of the transitions between the initial state J and the final state J′ using the Eq. (3). The probabilities of the radiative transitions are given by the equation:
The branching ratios can be to obtain from the probabilities of radiative transitions Arad by the equation:
The radiative lifetime of the level J is given by:
Another important term, integrated emission cross-section (in m), which is particularly useful to determine the possibility of lasing in glass, is defined as:
When the value of the integrated emission cross-section is close to or greater than ∼10−20 m, there is a possibility of lasing (Watekar et al., 2008).
The results obtained from the Judd-Ofelt analysis are reported in Tables 3–5.
Transition: 5I8→ |
λ(nm) | Oscillator strength f (×10−6) | |||||||||
---|---|---|---|---|---|---|---|---|---|---|---|
NPZS_H1 | NPZS_HY0.5 | NPZS_HY1 | NPZS_HY1.5 | NPZS_HY2 | |||||||
fmes | fcal | fmes | fcal | fmes | fcal | fmes | fcal | fmes | fcal | ||
5I7 | 1957 | 1.1951 | 1.30792 | 1.2529 | 1.5595 | 1.2983 | 1.5987 | 1.3064 | 1.8003 | 1.2161 | 1.4308 |
5I6 | 1155 | 0.5946 | 0.96128 | 0.6883 | 1.1498 | 0.6651 | 1.1765 | 0.6861 | 1.3338 | 0.6485 | 1.051 |
5F5 | 642 | 2.3739 | 2.57079 | 2.6415 | 3.0311 | 2.6752 | 3.1015 | 2.6738 | 3.3458 | 2.6391 | 2.8484 |
5F4 | 538 | 3.3668 | 2.79476 | 4.5804 | 3.3199 | 4.8053 | 3.4026 | 5.6358 | 3.7506 | 3.9579 | 3.0808 |
5F3 | 486 | 0.8187 | 1.04389 | 0.3176 | 1.2556 | 0.4405 | 1.2903 | 0.3395 | 1.4719 | 0.6037 | 1.1409 |
5F2, 3K8 | 474 | 0.5251 | 0.64620 | 0.6064 | 0.7773 | 0.1951 | 0.7987 | 0.3039 | 0.9112 | 0.4084 | 0.7063 |
5G6 | 448 | 12.4917 | 12.70179 | 13.331 | 13.625 | 12.587 | 12.869 | 13.473 | 13.743 | 13.006 | 13.292 |
5G5 | 418 | 2.3285 | 2.42021 | 2.5453 | 2.7951 | 2.5716 | 2.8468 | 2.5617 | 2.8813 | 2.5005 | 2.719 |
5G4 | 386 | 0.3533 | 0.32203 | 0.7069 | 0.3781 | 0.6512 | 0.3865 | 0.8467 | 0.4116 | 0.7537 | 0.3579 |
3H6 | 362 | 3.4714 | 2.37699 | 4.0711 | 2.5256 | 3.8578 | 2.3779 | 3.9563 | 2.5319 | 3.9731 | 2.484 |
RMS (×10−6) | ±0.511 | ±0.8946 | ±0.9261 | ±1.1160 | ±0.7466 |
Sample | Ω2 | Ω4 | Ω6 | Ω4/Ω6 | Trend |
---|---|---|---|---|---|
NPZS_H1 (present work) | 3.03 | 2.26 | 1.72 | 1.31 | Ω2 > Ω4 > Ω6 |
NPZS_HY0.5 (present work) | 3.13 | 2.62 | 2.07 | 1,26 | Ω2 > Ω4 > Ω6 |
NPZS_HY1(present work) | 2.83 | 2.66 | 2.12 | 1,25 | Ω2 > Ω4 > Ω6 |
NPZS_HY1.5(present work) | 3.09 | 2.69 | 2.42 | 1,11 | Ω2 > Ω4 > Ω6 |
NPZS_HY2(present work) | 3.07 | 2.54 | 1.88 | 1,35 | Ω2 > Ω4 > Ω6 |
Halogeno-phosphate (Bentouila et al., 2013) | 2.44 | 1.40 | 1.31 | 1.07 | Ω2 > Ω4 > Ω6 |
Phosphate (Reisfeld and Hormadaly, 1976) | 5.20 | 2.72 | 1.87 | 1.45 | Ω2 > Ω4 > Ω6 |
Germanate (Fan et al., 2015) | 4.70 | 1.63 | 0.81 | 2.01 | Ω2 > Ω4 > Ω6 |
Fluoride (Florez et al., 2006) | 1.56 | 3.72 | 2.86 | 1,30 | Ω2 < Ω6 < Ω4 |
Tellurite (Rai et al., 2003) | 4.98 | 0.99 | 2.96 | 0.33 | Ω4 < Ω6 < Ω2 |
Sample | Arad(s) | β(%) | Σ (×10−20 m) | τrad (ms) |
---|---|---|---|---|
NPZS_H1 (present work) | 57,357 | 1000 | 1519 | 17,435 |
NPZS_HY0.5 (present work) | 68.47 | 100 | 1.56 | 14.60 |
NPZS_HY1 (present work) | 70.21 | 100 | 1.60 | 14.24 |
NPZS_HY1.5 (present work) | 79.25 | 100 | 1.80 | 12.61 |
NPZS_HY2 (present work) | 63.00 | 100 | 1.43 | 15.87 |
Fluorophosphate (Tian et al., 2010) | 73.55 | 100 | – | 13.60 |
Germanate (Fan et al., 2011) | 97.84 | 100 | – | 10.22 |
Fluoride (Florez et al., 2006) | 109.93 | 100 | – | 9.09 |
4 Discussions
4.1 Thermal stability
Determination of glass transition temperature (Tg) and onset crystallization (Tx) is interesting for many reasons: in practical terms, Tg is a measure of approximate upper use temperature for a given composition, and annealing of glass samples is carried out somewhat below Tg. In addition, Tg is an important factor for laser glass. It is reported that a glass with high Tg has a good thermal stability to resist thermal damage at high pumping power (Xu et al., 2011a,b). Tx defines a safe upper limit for processing the melt if crystallization is to be avoided. From the DSC curves, one can observe that there is no crystallization peak till T = 450 ± 2°C for all samples, Tg is about 247 ± 2 °C and Tx is about 460 ± 2 °C for the glass sample without any doping ions and for glasses containing HoF3 and YbF3, the vitreous transition still situated around 250 °C and crystallization onset around 450 °C. One can notice that the glass transition temperature is shifted upwards high temperatures and the onset crystallization is shifted downwards low temperatures by addition of HoF3 and YbF3, results in agreement with previous works (Imanieh et al., 2014). As the changes of Tg and Tx is largely depending on the major mixing elements, because the temperature is closely related to the bonding force among the constituent elements, one can explain the shifting of Tg and Tx by structure arrangement of glass due to participation of Yb3+ and Ho3+ ions and enhanced glassy network structure (Chen et al., 2014). However, it has been observed that with high concentration of rare-earth, the glass became impossible to pour due to its high instability and during the pouring it could be crystallize to become opaque (Dantelle et al., 2005). Thus, an optimization of rare earth content is necessary to obtain a transparent glass.
The quantity ΔT = Tx − Tg, known as the supercooled liquid region, defined as the temperature gap between Tg and Tx. It has been used as a rough measure of the glass forming ability of a melt, i.e., of resistance against crystallization during casting (Wang, 2008). Large ΔT means strong inhibition to processes of nucleation and crystallization (Park et al., 2010; Xu et al., 2011a,b). For this reason, it is frequently used as an assessment criterion to determine the stability of glass. To achieve a large working range during operations such as fiber fabrication or the preparation of bulk glass articles, it is desirable to have ΔT as large as possible. We find that all glass samples have relatively a large value of ΔT (∼200 °C) close to the value obtained for fluorophosphate glasses (Tian et al., 2010; Chen et al., 2014), which is significantly higher than that of other kinds of rare earth doped fluorophosphate glasses such as Tm3+ (Liao et al., 2007). This result means that all our glass samples are stable against crystallization.
From the analysis of DSC curve, we can conclude that thermal properties of our glass samples are good for fiber drawing.
4.2 Absorption spectra and Judd-Ofelt analysis
Absorption spectra of the Ho3+ and Ho3+/Yb3+ co-doped glass samples are shown in Fig. 3. The spectroscopic properties of our glass samples were determined using the Judd-Ofelt model. The f-f transitions are considered to be electric dipole in nature, because the magnetic dipole oscillator strength (fmd) will be relatively small (Sooraj Hussain et al., 2006). For that reason in the present work, these magnetic dipole line strengths have not been considered. Measured and calculated oscillator strength for Ho3+ ions in NPSZ_HY samples were presented in Table 3.The low RMS values suggest the good agreement between calculated and experimental oscillator strengths of Ho3+ ions in our glasses samples which indicates the validity of the Judd-Ofelt model for predicting the spectroscopic properties of Ho3+.
The Judd-Ofelt parameters Ωk (k = 2, 4, 6) are mainly depend on the host glass composition. These parameters can provide versatile information regarding the rare earth in glass structure. Some empirical correlations of the Judd-Ofelt parameters and the local structure of the rare earth ions have been reported in literature (Jacobs and Weber, 1976; Ebendorff-Heidepriem et al., 1998; Malta and Carlos, 2003). Generally, Ω2 is an indicator of the covalency of the rare earth-ligand bonds (short-range effect), and it is hypersensitive to the compositional changes in the host materials. Ω2 is also related with the symmetry of ligand field in the glass host. According to previous studies (Ebendorff-Heidepriem and Ehrt, 1999), Ω2 increase with the increasing of covalency and asymmetry at the rare earth sites. The values for Ω4 and Ω6 provide some information of the rigidity of the host materials (Wang, 2008). They depend on bulk properties such as viscosity and dielectric constant of the media (long-range effects). They are also affected by the vibronic transitions of the rare earth ions bound to the ligand atoms. In the present work, the Judd-Ofelt parameters Ωλ are calculated and compared with those of various Ho3+ doped glasses, the results were presented in Table 4. One can observe, from the values of Ω2 of our samples, that the covalent environment for Ho3+ is slightly decreased with increasing of Yb3+ amount in glasses. The observed Ω2 values of our samples are located between the higher side to the values reported for ionic glasses (for fluoride glasses, Ω2 ∼ 2 × 10−20 cm2) and those of the covalent glasses (for phosphates Ω2 ∼ 5 × 10−20 cm2). Theoretically, the polarizability of oxide ions is higher than that of fluorine ions, resulting in the increasing covalence of the bonds between rare earth ions and surrounding ligand from fluoride to phosphate glasses due to substitution of fluorine ions by oxygen ions. As fluorophosphate glasses has both O2− and F− ions, the value of Ω2 is larger than that of fluoride glass and smaller than that of phosphate glass (Tian et al., 2010). However, the observed lower values of Ω2 parameter for Ho3+/Yb3+ co-doped NPSZ_HY glasses compared by those of phosphate glasses indicates that the ligand asymmetry around the rare earth ions in Ho3+/Yb3+ co-doped NPSZ_HY glasses is weaker than those in phosphate glasses. Additionally, a larger modifier ion in phosphate glass gives rise to a larger average between P-O-P chains causing the increasing average Ho-O distance to increase. Such increase in the bond lengths produces weaker local field near rare earth ions and lead to lower value of Ω2 (Rao et al., 2012).
According to the theory of Jacobs and Weber (Jacobs and Weber, 1976), the rare earth emission intensity can be characterized uniquely by Ω4 and Ω6 parameters. Thus, we used the so-called spectroscopic quality factor (Ω4/Ω6). This factor is important in predicting the behavior of various lasing transitions in a given matrix. Based on this factor, it is found that the NPZS_HYy glasses appear to be better optical glasses. Moreover, it is noticed that the values of Judd-Ofelt parameters of our sample glasses follows the trend Ω2 > Ω4 > Ω6 in consistent with that one observed for halogeno-phosphate, phosphate and germanate glasses; nevertheless, it differs from those of fluoride and tellurite glasses.
Table 5 presents the predicted radiative transition probability Ar, branching ratios β, integrated emission cross-section and radiative lifetimes τrad for 5I7 → 5I8 transition of Ho3+ ions in NPSZ_HY samples. The results show that the values Arad of the 5I7 level of Ho3+ were 68.4, 70.2, 79.2 and 63.0 s−1, which are similar to the value found for fluorophosphates glass and smaller than that of germanate and fluoride glasses. It is reasonable to obtain such result because the radiative transition probability is proportional to n(n2 + 2)2/9 (Eq. (5) in Section 3.3) and the refractive index of fluorophosphates glasses is usually less then germanate and fluoride glasses. It is evident to find also that the radiative lifetimes of the 5I7 level of Ho3+ for NPZS_HYy glasses are greater than those of germanate and fluoride glasses. Further, the radiative lifetimes values for Ho3+/Yb3+ co–doped NPZS_HYy glasses are found to be smaller than that for individually Ho3+ doped NPZS_H1 glass. This result, which may be explained by the crucial contribution of phonon subsystem, is in agreement with those found by Gandhi et al. (2010) for the measured lifetime of blue, green and red transitions.
The values of the integrated emission cross-sections obtained are greater than ∼10−20 m, which indicates a possibility of lasing with these glasses. But it must be mentioned that lasing can be specifically determined only if emission cross-section and fluorescence lifetime are taken into account.
5 Conclusions
A series of Ho3+/Yb3+ co-doped new fluorophosphate glasses have been investigated and characterized. All the prepared samples exhibit a high thermal stability against crystallization compared with other kinds of rare earth doped fluorophosphate glasses. From the absorption spectra, Judd-Ofelt parameters are obtained and discussed. The spectroscopic properties of Ho3+ in these glasses, such as radiative transition probabilities, integrated emission cross-sections and radiative lifetimes, were calculated and compared with other glasses. Spectroscopic quality factor (Ω4/Ω6) and the integrated emission cross-section were found greater than 1 and about 10−20 m respectively, which indicates better properties of these glasses as lasing materials. Ho3+/Yb3+ co–doped fluorophosphate glasses have predicted radiative lifetimes smaller than that for individually Ho3+ doped fluorophosphate glass which may be explained by the crucial contribution of phonon subsystem. Consequently, these results indicate these glasses appear as a potential lasing materials and offer prospects for photonics applications.
References
- Rare-earth-doped chalcogenide glasses for mid-IR gas sensor applications. Proc. SPIE. 2017;10100:101000Q.
- [CrossRef] [Google Scholar]
- Emission characteristics of holmium ions in fluoro-phosphate glasses for photonic applications. AIP Conf. Proc.. 2016;1731:1-4.
- [CrossRef] [Google Scholar]
- Light emission at 2 μm from Ho-Tm-Yb doped silicate glasses. Opt. Mater. (Amst). 2011;33:1316-1319.
- [CrossRef] [Google Scholar]
- Spectroscopic studies of rare earth-doped halogeno-phosphate glasses. J. Optoelectron. Adv. Mater.. 2013;15
- [Google Scholar]
- Spectral intensities of the trivalent lanthanides and actinides in solution. I. Pr 3+, Nd 3+, Er 3+, Tm 3+, and Yb 3+. J. Chem. Phys.. 1965;42:3797-3806.
- [CrossRef] [Google Scholar]
- Spectral intensities of the trivalent lanthanides and actinides in solution. II. Pm 3+, Sm 3+, Eu 3+, Gd 3+, Tb 3+, Dy 3+, and Ho 3+. J. Chem. Phys.. 1968;49:4412-4423.
- [CrossRef] [Google Scholar]
- Ho3+ doped fluorophosphate glasses sensitized by Yb3+ for efficient 2 μm laser applications. Opt. Commun.. 2014;321:183-188.
- [CrossRef] [Google Scholar]
- Thermal and luminescent properties of 2 μm emission in thulium-sensitized holmium-doped silicate-germanate glass. Photonics Res.. 2016;4:214-221.
- [CrossRef] [Google Scholar]
- Costa, F.B., Yukimitu, K., Nunes, L.A.O., Andrade, L.H. da C., Sandro Marcio Lima, J.C., Moraes, S., 2015. Characterization of Nd3 + -doped Tellurite Glasses with Low OH Content. Mater. Res. 18;2–7. https://doi.org/10.1590/1516-1439.320614.
- Nucleation efficiency of erbium and ytterbium fluorides in transparent oxyfluoride glass-ceramics. J. Mater. Res.. 2005;20:472-481.
- [CrossRef] [Google Scholar]
- Spectroscopic properties and design of highly luminescent lanthanide coordination complexes. Coord. Chem. Rev. 2000
- [CrossRef] [Google Scholar]
- Spectroscopic study of Sm: Ce ions co-doped in barium fluoroborate glass. J. Non. Cryst. Solids. 2010;356:1650-1654.
- [CrossRef] [Google Scholar]
- Effect of glass composition on Judd-Ofelt parameters and radiative decay rates of Er3+ in fluoride phosphate and phosphate glasses. J. Non. Cryst. Solids. 1998;240:66-78.
- [CrossRef] [Google Scholar]
- Tb3+ f–d absorption as indicator of the effect of covalency on the Judd-Ofelt Ω2 parameter in glasses. J. Non. Cryst. Solids. 1999;248:247-252.
- [CrossRef] [Google Scholar]
- Spectroscopic properties and energy transfer in Yb3+–Ho3+ co-doped germanate glass emitting at 2.0 μm. J. Non. Cryst. Solids. 2011;357:2431-2434.
- [CrossRef] [Google Scholar]
- Spectroscopic properties and quenching mechanism of 2 µm emission in Ho3+ doped germanate glasses and fibers. Opt. Mater. Express. 2015;5:1356-1365.
- [CrossRef] [Google Scholar]
- Spectroscopic characterization of Ho3+ ion-doped fluoride glass. J. Alloys Compd.. 2006;418:238-242.
- [CrossRef] [Google Scholar]
- Rare-earth doped fluoride phosphate glasses: structural foundations of their luminescence properties. Phys. Chem. Chem. Phys.. 2017;19:21612-21624.
- [CrossRef] [Google Scholar]
- Near-IR emission from holmium-ytterbium co-doped alkali bismuth gallate and fluorophosphate fiber glass preforms. J. Alloys Compd.. 2009;473:500-504.
- [CrossRef] [Google Scholar]
- Influence of aluminum ions on fluorescent spectra and upconversion in codoped CaF2-Al2O3-P2O 5-SiO2: Ho3+ and Er3+ glass system. J. Appl. Phys.. 2010;108
- [CrossRef] [Google Scholar]
- A review of recent progress in holmium-doped silica fibre sources. Opt. Fiber Technol.. 2014;20:621-630.
- [CrossRef] [Google Scholar]
- Structural and optical studies of Yb3+, Er3+ and Er3+/Yb3+ co-doped phosphate glasses. J. Rare Earths. 2013;31:685-693.
- [CrossRef] [Google Scholar]
- Imanieh, M.H., Martín, I.R., Gonzalez-Platas, J., Eftekhari Yekta, B., Marghussian, V.K., Shakhesi, S., 2014. Behavior of Yb 3+ and Er 3+ during Heat Treatment in Oxyfluoride Glass Ceramics 2014. https://doi.org/10.1155/2014/171045.
- Dependence of the 4 F 3/2→ 4 I 11/2 induced-emission cross section for Nd 3+ on glass composition. IEEE J. Quantum Electron.. 1976;12:102-111.
- [CrossRef] [Google Scholar]
- Optical absorption intensities of rare-earth ions. Phys. Rev.. 1962;127:750-761.
- [CrossRef] [Google Scholar]
- Recent developments in rare-earthdoped materials for optoelectronics. Prog. Quant. Elec.. 2002;26:225-284.
- [CrossRef] [Google Scholar]
- GeS2–In2S3–CsI Chalcogenide Glasses Doped with Rare Earth Ions for Near- and Mid-IR Luminescence. Sci. Rep.. 2016;6:37577.
- [CrossRef] [Google Scholar]
- Stability against crystallization and spectroscopic properties of Tm3+ doped fluorophosphate glasses. Opt. Mater. (Amst). 2007;29:867-872.
- [CrossRef] [Google Scholar]
- Intensities of 4f–4f transitions in glass materials. Quim. Nova. 2003;26:889-895.
- [CrossRef] [Google Scholar]
- Meruva, S., Carlos, B.L., Alberto Peres, F.J.J., 2014. Optical characterization, luminescence properties of Er 3+ and Er 3+ /Yb 3+ co-doped tellurite glasses for broadband amplification 8961, 896132. https://doi.org/10.1117/12.2037000.
- Rare-earth doped oxyfluoride glass-ceramics and fluoride ceramics: Synthesis and optical properties. Opt. Mater. (Amst). 2007;29:1263-1270.
- [CrossRef] [Google Scholar]
- Intensities of Crystal Spectra of Rare-Earth Ions. J. Chem. Phys.. 1962;37:511-520.
- [CrossRef] [Google Scholar]
- Impact of ytterbium on photoluminescence from the modifier in TeO2-ZnO:Ho3+ glass. Phys. B Condens. Matter. 2016;480:137-140.
- [CrossRef] [Google Scholar]
- Abnormal behavior of supercooled liquid region in bulk-forming metallic glasses. J. Appl. Phys.. 2010;108
- [CrossRef] [Google Scholar]
- Spectroscopic properties of Ho3+ ions doped in tellurite glass. Spectrochim. Acta Part A Mol. Biomol. Spectrosc.. 2003;59:3221-3226.
- [CrossRef] [Google Scholar]
- Role of modifier oxide in emission spectra and kinetics of Er-Ho codoped Na 2SO 4-MO-P 2O 5 glasses. Spectrochim. Acta - Part A Mol. Biomol. Spectrosc.. 2012;86:472-480.
- [CrossRef] [Google Scholar]
- Prospects of Holmium-doped fluorides as gain media for visible solid state lasers. Opt. Mater. Express. 2015;5:88.
- [CrossRef] [Google Scholar]
- Optical intensities of holmium in tellurite, calibo, and phosphate glasses. J. Chem. Phys.. 1976;64:3207-3212.
- [CrossRef] [Google Scholar]
- Efficient Nd3+→Yb3+ energy transfer processes in high phonon energy phosphate glasses for 1.0 μm Yb3+ laser. J. Appl. Phys.. 2011;109:1-11.
- [CrossRef] [Google Scholar]
- Tunable 2 µ m ZrO 2 –Y 2 O 3 –Ho 2 O 3 solid-state laser. Laser Phys. Lett.. 2017;14:55807.
- [CrossRef] [Google Scholar]
- NIR emission studies and dielectric properties of Er3+-doped multicomponent tellurite glasses. Spectrochim. Acta Part A Mol. Biomol. Spectrosc.. 2016;161:130-137.
- [CrossRef] [Google Scholar]
- Role of Al coordination in barium phosphate glasses on the emission features of Ho3+ion in the visible and IR spectral ranges. J. Lumin.. 2010;130:498-506.
- [CrossRef] [Google Scholar]
- Spectroscopic properties of Ho 3+, Tm 3+, and Ho 3+ /Tm 3+ doped tellurite glasses for fiber laser applications. Proc. SPIE. 2014;8961:896139.
- [CrossRef] [Google Scholar]
- Absorption and emission properties of Ho3+ doped lead-zinc-borate glasses. Thin Solid Films. 2006;515:318-325.
- [CrossRef] [Google Scholar]
- 2 μm Emission of Ho3+-doped fluorophosphate glass sensitized by Yb3+. Opt. Mater. (Amst). 2010;32:1508-1513.
- [CrossRef] [Google Scholar]
- Investigation of thermal stability and spectroscopic properties in Er3+/Yb3+ co-doped niobic tungsten tellurite glasses. Spectrochim. Acta Part A Mol. Biomol. Spectrosc.. 2008;70:99-103.
- [CrossRef] [Google Scholar]
- Enhanced 2 μm emission of Yb-Ho doped fluorophosphates glass. J. Non. Cryst. Solids. 2011;357:2447-2449.
- [CrossRef] [Google Scholar]
- Optical properties of Ho-doped alumino-germano-silica glass optical fiber. J. Non. Cryst. Solids. 2008;354:1453-1459.
- [CrossRef] [Google Scholar]
- 2μm spectroscopic investigation of Tm3+-doped tellurite glass fiber. J. Non. Cryst. Solids. 2011;357:2489-2493.
- [CrossRef] [Google Scholar]
- Broadband 2 μm emission and energy-transfer properties of thulium-doped oxyfluoride germanate glass fiber. Appl. Phys. B Lasers Opt.. 2011;104:839-844.
- [CrossRef] [Google Scholar]
- Żmojda, J., Dorosz, D., Kochanowicz, M., Miluski, P., Dorosz, J., 2012. Yb 3+ /Ho 3+ -codoped antimony-silicate optical fiber 8454, 845414. https://doi.org/10.1117/12.2000174.