Molecular characterization of biosurfactant producing marine bacterium isolated from hydrocarbon-contaminated soil using 16S rRNA gene sequencing
⁎Corresponding author. n_arunagiri@yahoo.co.in (Narasingam Arunagirinathan)
-
Received: ,
Accepted: ,
This article was originally published by Elsevier and was migrated to Scientific Scholar after the change of Publisher.
Peer review under responsibility of King Saud University.
Abstract
Objectives
This study was aimed to isolate and identify the biosurfactant producing marine bacteria from petroleum hydrocarbons contaminated sediments by 16S rRNA gene sequencing.
Methods
Soil sample was enriched with diesel (2%) as the sole source of carbon in minimal salt medium for 3 weeks at 28 °C ± 2. Enriched sample was plated on nutrient agar and the organisms are selected based on the colony morphology. Biosurfactant producing efficiency of the isolates was assessed using blood haemolysis, oil displacement test, microplate assay (drop collapse test) and emulsification activity. Identification of the efficient biosurfactant producing isolate was done by gram staining and biochemical tests. Molecular characterization was done using 16S rRNA gene sequencing.
Results
Totally seven isolates were selected based on colony morphology and among them, the isolate ADY2b was able to reduce surface tension significantly in the oil displacement test and also formed a stable emulsion. The emulsification index (E24) had shown a promising result of 58.33%. The isolate ADY2b was a motile, gram negative rod shaped bacterium and positive for catalase and oxidase. Sequence alignment of the 16S rRNA of the ADY2b strain and database search revealed 98% similarity to Pseudomonas mendocina by BLAST analysis.
Conclusion
Seven bacteria were isolated from petroleum hydrocarbon contaminated sediments. Strain ADY2b had shown promising results on the production of biosurfactants and it is capable of increasing the bioavailability of poorly soluble petroleum hydrocarbons. The isolate Pseudomonas mendocina ADY2b obtained in the study had potential to be used in oil degradation purposes.
Keywords
Biosurfactants
Petroleum hydrocarbons
16S rRNA
Microplate assay
Emulsification activity (E24)
Pseudomonas species
1 Introduction
Surfactants, are “surface-active-agents”, basically chemical compounds which lower the surface tension of a liquid, the interfacial tension between two liquids, or that between a liquid and a solid. Surfactants are amphiphilic compounds that reduce the free energy of the system by replacing the bulk molecules of higher energy at an interface (Mulligan, 2005). They contain a hydrophobic moiety with little affinity for the bulk medium and a hydrophilic portion that is attracted to the bulk medium. Surfactants have been used industrially as adhesives, emulsifies, flocculating, wetting and foaming agents, lubricants and penetrants (Mulligan and Gibbs, 1993). Because of their amphiphilic nature, surfactants tend to accumulate at interfaces (air–water and oil–water) and surfaces. As a result, surfactants reduce the forces of repulsion between unlike phases at interfaces or surfaces and allow the two phases to mix more easily (Bodour and Miller-Maier, 1998). Biosurfactants are polymers, totally or partially extracellular, amphipathic molecules containing polar and non polar moieties which allow them to form micelles that accumulate at interphase between liquids of different polarities such as water and oil, thereby reducing surface tension and facilitating hydrocarbon uptake and emulsification (Desai and Banat, 1997; Thavasi et al., 2009). The superior properties of biosurfactants include high biodegradability, low toxicity, ecological acceptability, and production from cheaper substrates (Nitschke and Pastore, 2004). Biosurfactants are widely used in hydrocarbon bioremediation field since they can enhance the growth on hydrophobic surface and can increase the nutrient uptake of hydrophobic substrates thereby overcoming the poor availability of hydrocarbon contaminants to microorganisms (Canet et al., 2001; Sotivora et al., 2009). Extracellular excretion of biosurfactants by bacteria has been reported by many studies (Pornsunthorntawee et al., 2007; Kumar et al., 2008; Thavasi et al., 2008). Among the various bacterial species, Pseudomonas species are the best known for degrading hydrocarbon and producing biosurfactants mainly rhamnolipid in nature (Rahman et al., 2002). Oil residues in the marine environment create a major risk to the aquatic animals and also to humans through food chain. Hence, the identification of good biosurfactant producing environmental bacterium is needed. To probe this, the study was aimed to isolate and characterize the most efficient biosurfactant producing bacteria from petroleum hydrocarbon contaminated environmental samples.
2 Materials and methods
2.1 Isolation of biosurfactant-producing marine bacteria by enrichment method
The sediment samples were collected from 6 spots with the designation SAN1, ADY2, KLM3, PRG4, TRP5 and KTR6 in and around the coastal line of Chennai harbor having an exposure history of crude oil, white oil and furnace oil since 1972 when the first oil terminal was commissioned. Sediments were collected in sterile polypropylene containers and transported to the laboratory within 2 h and stored at 4 °C until further process. Four samples were collected in different areas of each spot, and all four samples were pooled to make a single sample. The samples collected were shade dried, sieved and homogenized. Enrichment was done in minimal salt medium (MSM) with 2% diesel as the sole source of carbon in shaking condition at 150 rpm with constant transfer to fresh medium at an interval of 7 days for 3 weeks. Then the samples were spread plated on nutrient agar (Difco, USA) (pH 7.2 ± 0.2) prepared in sterilized seawater and incubated at 28 °C ± 2 for 1–2 days. After incubation, morphologically different colonies were selected and purified by repeated streaking and stored as slant cultures at 4–8 °C and glycerol stock (30%) at −80 °C for further studies (Maneerat and Phetrong, 2007).
2.2 Biosurfactant activity assays
2.2.1 Haemolytic activity
Isolated strains were screened on blood agar plates containing 5% (v/v) sheep blood and incubated at room temperature for 24 h. Haemolytic activity was detected as the occurrence of a defined clear zone (alpha, beta and gamma haemolysis) around a bacterial colony.
2.2.2 Oil displacement test
Oil displacement is a method used to determine the diameter of the clear zone, which occurs after adding surfactant-containing solution on an oil–water interphase. The diameter evaluation allows the surface tension reduction efficiency of a given biosurfactant. 15 ml distilled water was added to a petriplate which is 90 mm in diameter. 100 μl of diesel was added to the water surface, followed by the addition of 20 μl of cell culture supernatant onto the oil surface. The diameter of the clear halo visualized under visible light was measured after 30 s (Rodrigues et al., 2006). Sodium dodecyl sulfate (SDS) is an effective surfactant used as positive control and culture uninoculated MSM was used as negative control.
2.2.3 Microplate assay (Drop collapse test)
The surface activity of individual strain was determined qualitatively with the microtitre plate assay (Vaux and Cottingham, 2001). 100 μl of the culture supernatant was added into a microwell of a 96-mircowell plate coated with diesel. The plate was viewed using a backing sheet of paper with a grid. If biosurfactant is present, the concave surface distorts the image of the grid below. The optical distortion of the grid provides a qualitative assay for the presence of surfactants.
2.2.4 Emulsification index (E24)
Emulsification Index of the biosurfactant was done using diesel (Cooper and Goldenberg, 1987). A mixture of 3 ml of hydrocarbon and 3 ml of cell free extract obtained after the centrifugation of sample culture was taken in a test tube and homogenized by vortexing for 2 min. The emulsion activity was investigated after 24 h and the emulsification index (E24) was calculated by the height of the emulsion by the total height of the aqueous layer and then multiplying by 100.
2.3 Identification of strains and DNA extraction
Preliminary identification of the isolate was carried out using gram staining, motility and biochemical tests using conventional bacterial culture identification methods. The single pure colonies of the bacterial isolates were grown in Luria-Bertani (LB) broth overnight at 28 °C. 2 ml of the culture was centrifuged at 5000 rpm for 5 min and pellet was suspended in 200 µl of TE (pH = 8) containing RNase (50 ng /ml), then 400 µl of lysis buffer was added followed by mixed well and incubated for 15 min at 37 °C with intermittent shaking for every 5 min. Immediately chloroform and isoamylalcohol in the ratio (24:1) was taken and mixed by inversion. Tubes were centrifuged at 10000 rpm for 5 min, supernatant was transferred carefully to another microcentrifuge tube. To the supernatant, 0.1 vol 3 M sodium acetate (pH = 5.2) and 0.6 vol isopropanol were added, mixed well by inversion and kept in the ice for 10 min followed by centrifugation at 1000 rpm for 10 min. The pellet was washed with 70% ethanol with gentle shaking and centrifuged at 10000 rpm for 3 min. Supernatant was removed and pellet was air dried. Extracted DNA was visualized using 0.8% agarose gel electrophoresis and images were documented.
Using 16S ribosomal RNA gene specific universal primers 27F 5′-AGA GTT TGA TCC TGG CTC AG-3′ and 1492R 5′-GGT TAC CTT GTT ACG ACT T-3′ (Sigma), the 16S rRNA gene was amplified with 50 μl reaction mixture containing 1X reaction buffer (10 mM Tris [pH 8.3], 50 mM KCl and 1.5 mM MgCl2), 200 μl dNTPs, 0.05 U Taq DNA polymerase enzyme (Sigma, USA), 0.5 μM of each primer and 1 ng template DNA. The thermal cycling conditions were: 5 min at 94 °C for initial denaturation; 31 cycles of 30 s at 95 °C, 1 min at 54 °C, 2 min at 72 °C, and a final extension for 5 min at 72 °C. The amplification reaction was performed with a thermal cycler (MyCycler, Bio-Rad, USA) and the PCR amplicons (approximately 1500 bp) were resolved by electrophoresis in 1% (w/v) agarose gel to confirm the expected size of the product.
2.4 Phylogenetic analysis
The 16 rRNA gene sequence obtained in this study was aligned with the sequences published in NCBI. Aligned sequences were edited and phylogenetic tree was constructed using MEGA6 software. The phylogenetic tree for relationship among strains was constructed by the Maximum Liklihood Method with a boot-strap of 500 using Kimura-2 parameter.
3 Results and discussion
3.1 Enrichment and isolation of the bacterial isolates
Hydrocarbon contaminated sediment samples were collected from six different sites in and around the coastal line of Chennai harbour and designated as SAN1, ADY2, KLM3, PRG4, TRP5 and KTR6. They were kept for enrichment in Mineral Salt Medium (MSM) with 2% diesel as the sole source of carbon at 28 °C ± 2 for 21 days with constant shaking. Enriched samples were serially diluted and spread plated on nutrient agar amended with diesel oil. Morphologically seven distinct colonies (flat, large, irregular, opaque, light green pigmented, and non-pigmented) were selected, pure cultures were obtained and designated as SAN1a, ADY2a, ADY2b, KLM3c, PRG4a, TRP5a, KTR6b. The seven isolates were further subjected to screening of biosurfactants.
3.2 Screening for biosurfactant production
In the present study all the isolates were subjected to different types of screening methods given by Satpute et al. (2008) Among seven bacterial isolates, only four isolates (ADY2b, KTR6b, KLM3c and PRG4a) produced β-haemolysis. The remaining isolates did not show any haemolysis and the results were tabulated (Table. 1). Blood haemolysis has been chosen to be the preliminary screening method for biosurfactant production (Ghayyomi Jazeh et al., 2012). Safary et al. (2010) reported that while the other microbial products such as virulence factors lyse blood agar, the biosurfactants that are poorly diffusible will not lyse blood cells. However, blood agar lysis screening can be used as a rapid screening method, in which samples with the positive result are subsequently subjected to biosurfactant-activity tests in liquid media. In this study the isolates ADY2b, KLM3c, PRG4a and KTR6b that had shown complete haemolysis were subjected to further analysis for biosurfactant production.
Isolates | Blood haemolysis | Oil displacement test | Microplate assay | Emulsification Index (E24) (%) |
---|---|---|---|---|
SAN1a | No haemolysis | + | – | – |
ADY2a | No haemolysis | – | – | – |
ADY2b | β-haemolysis | + | + | 58.33 |
KLM3c | β-haemolysis | + | – | 26.66 |
PRG4a | β-haemolysis | + | – | 23.33 |
TRP5a | No haemolysis | – | – | – |
KTR6b | β-haemolysis | + | – | 30.00 |
Note: + = Positive, − = Negative.
The micro-organisms which produce biosurfactant can only displace the oil. The drop collapse test and oil displacement tests are indicative of the surface and wetting activities (Youssef et al., 2004). Oil displacement test is an indirect measurement of surface activity of a sample tested against diesel oil, a larger diameter represents a higher surface activity of the testing solution (Rodrigues et al., 2006). In oil displacement test all the four isolates, that had shown blood haemolysis, displaced the oil and produced zones showing various diameters and the results were tabulated (Table 1). The isolate ADY2b produced a clear zone of 3.7 cm showing better activity than the strain CpA 1 isolated by Safary et al. that had shown a zone of 3.1 cm. The halo zones formed by culture supernatants of the four isolates that were measured in centimetres are given graphically (Fig. 2). In microplate assay (drop collapse test) isolate ADY2b was the only strain to give positivity which is an indicative of biosurfactant production (Table 1 & Fig. 1a). The growth of bacteria on hydrocarbons is usually accompanied by the production of surfactants that helps in the adherence of the cells to oil droplets (Rosenberg and Rosenberg, 1981).
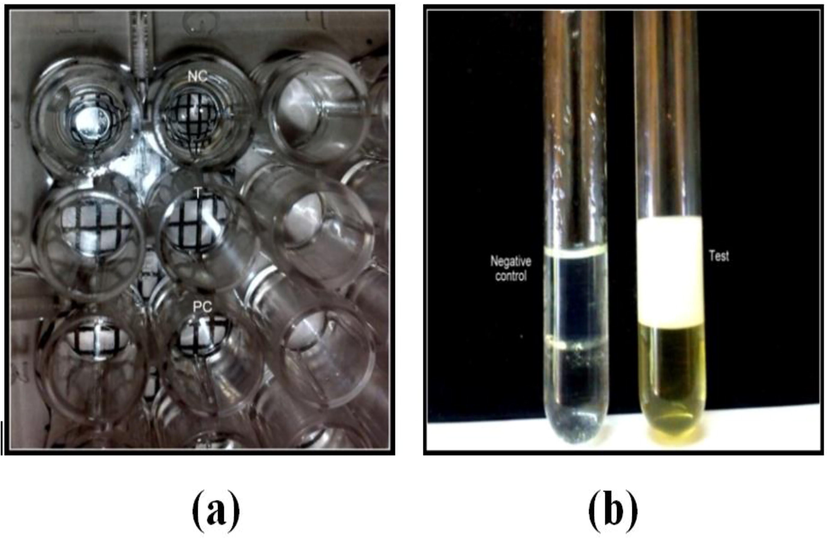
- (a) Showing microplate test & (b) Emulsification Index (E24).
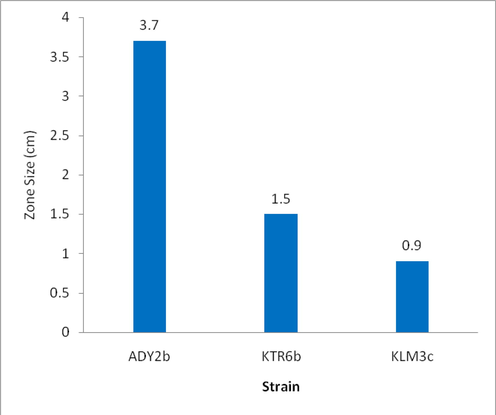
- Showing the zone size produced by bacterial isolates in the oil displacement test.
Emulsification index determines the productivity of bioemulsifiers (Bonilla et al., 2005). Thus, by examining the emulsification units, it is possible to select a potent biosurfactant/bioemulsifier producer and this is one of the important and effective assays for screening the biosurfactant/bioemulsifier producers as reported by Satpute et al. (2008). Strain ADY2b had shown better emulsification index of about 58.33% (Table 1 & Fig. 1b) when compared with that of Bacillus subtilis (20%) and Bacillus cereus (30%) on diesel oil reported by Jayasree et al. (2011). The emulsification index (E24) is given in Table 1. Since ADY2b had shown promising results for all the tests performed for biosurfactant production, this isolate was further subjected to microscopical, biochemical and molecular characterization.
3.3 Identification of the potent isolate
Microscopic and biochemical studies on the isolate ADY2b indicated that it was Gram-negative, motile, non-spore forming rod and had shown positive for catalase and oxidase production. Based on the above results, this strain was preliminarily identified as Pseudomonas sp. The genomic DNA of ADY2b (approximately 4–5 Mb) was extracted and confirmed through agarose gel electrophoresis. The 16S rRNA of the strain was amplified and sequenced. Sequencing results showed the gene size of 1441 base pairs. Sequence alignment of the 16S rRNA gene of the strain and Data base search revealed 98% similarity to Pseudomonas mendocina by BLAST analysis and the identified gene was submitted in the GenBank (Accession Number-KF378590). The phylogenetic tree was constructed with the data obtained through the BLAST results (Fig. 3). In the present study, the bacterial strain ADY2b is identified as Pseudomonas mendocina ADY2b which throws light on the fact that Pseudomonas species are the major group of organisms colonizing the hydrocarbon contaminated sites in the environment as reported by Sneha et al. (2012).
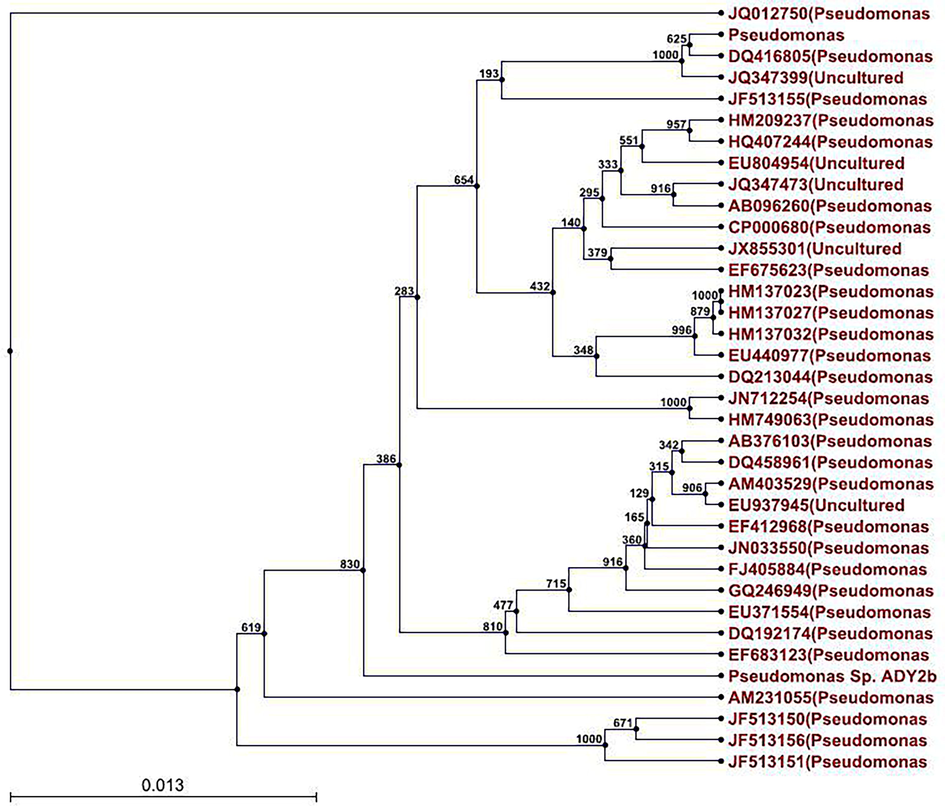
- Phylogenetic tree showing the position of the bacterial isolate ADY2b within the Pseudomonas group, constructed with Maximum-Likelihood Method.
4 Conclusion
Very few studies have been reported on the use of marine bacteria for the production of biosurfactants and bioremediation of oil contaminated sites. In this study, seven bacterial strains were isolated from petroleum hydrocarbon contaminated sediments. Strain ADY2b had shown promising results on the production of biosurfactants and it is capable of increasing the bioavailability of poorly soluble petroleum hydrocarbons. Results of the present study suggested that the isolate Pseudomonas mendocina ADY2b has potential for its usage in oil degradation purposes. Biosurfactant producing capacity of this strain can be further increased by optimization studies.
Acknowledgement
The authors extend their appreciation to the Researchers Supporting Project number RSP2022R470, King Saud University, Riyath, Saudi Arabia.
Declaration of Competing Interest
The authors have none to declare in this aspect.
References
- Application of a modified drop-collapse technique for surfactant quantitation and screening of biosurfactant-producing microorganisms. J. Microbiol. Met.. 1998;32(3):273-280.
- [CrossRef] [Google Scholar]
- Production and characterization of a new bioemulsifier from Pseudomonas putida ML2. J. Appl. Microbiol.. 2005;98(2):456-463.
- [CrossRef] [Google Scholar]
- Biodegradation of polycyclic aromatic hydrocarbons (PAHs) by native microflora and combination of white rot fungi in coal-tar contaminated soil. Biores. Technol.. 2001;76:113-117.
- [CrossRef] [Google Scholar]
- Surface active agents from two Bacillus species. Appl. Environ. Microbiol.. 1987;53(2):224-229.
- [Google Scholar]
- Microbial production of biosurfactants and their commercial potential. Microbiol. Mol. Biol. Rev.. 1997;61:41-64.
- [Google Scholar]
- Biosurfactant production by Bacillus sp isolated from petroleum contaminated soils of Sirri Island. Am. J. Appl. Sci.. 2012;9:1-6.
- [CrossRef] [Google Scholar]
- Isolation of biosurfactant producing bacteria from environmental samples. Pharmacologyonline. 2011;3:1427-1433.
- [Google Scholar]
- Biosurfactant production and hydrocarbon degradation by halotolerant and thermotolerant Pseudomonas sp. World. J. Microbiol. Biotechnol.. 2008;24:1047-1057.
- [CrossRef] [Google Scholar]
- Isolation of biosurfactant-producing marine bacteria and characteristics of selected biosurfactant. Songklanakarin J. Sci. Technol.. 2007;29:781-791.
- [Google Scholar]
- Environmental applications for biosurfactants. Environ. Poll.. 2005;133(2):183-198.
- [CrossRef] [Google Scholar]
- Factors influencing the economics of biosurfactants. In: In Biosurfactants: Production, Properties, Applications. Vol 48. Boca Raton, Florida, US: CRC Press; 1993. p. :329-371.
- [Google Scholar]
- Biosurfactant production by Bacillus subtilis using cassava processing effluent. Appl. Biochem. Biotechnol.. 2004;112:163-172.
- [CrossRef] [Google Scholar]
- Structural and physiochemical characterization of crude biosurfactant produced by Pseudomonas aeruginoa SP4 isolated from petroleum contaminated soil. Biores. Technol.. 2007;99:1589-1595.
- [CrossRef] [Google Scholar]
- Bioremediation of gasoline contaminated soil by a bacterial consortium amended with poultry litter, coir pith, and rhamnolipid biosurfactant. Biores. Technol.. 2002;81:25-32.
- [CrossRef] [Google Scholar]
- Physiochemical and functional characterization of a biosurfactant produced by Lactococcus lactis 53. Colloid Surface B. 2006;49:79-86.
- [CrossRef] [Google Scholar]
- Role of adherence in growth of Acinetobacter calcoaceticus RAG-1 on hexadecane. J. Bacteriol.. 1981;148:51-57.
- [CrossRef] [Google Scholar]
- Isolation and characterization of biosurfactant producing bacteria from Caspian Sea. Biotechnol.. 2010;9(3):378-382.
- [CrossRef] [Google Scholar]
- Assessment of different screening methods for the selecting biosurfactant producing marine bacteria. Indian J. Marine Sci.. 2008;37:243-250.
- [Google Scholar]
- Isolation and screening of biosurfactants produced by Pseudomonas aeruginosa from oil spilled soils. Int. J. Pharm. Biol. Arch.. 2012;3:321-325.
- [Google Scholar]
- Effect of rhamnolipid biosurfactant on cell surface of Pseudomonas aeruginosa. Microbiol. Res.. 2009;164:297-303.
- [CrossRef] [Google Scholar]
- Production and characterization of a glycolipid biosurfactant from Bacillus megaterium using economically cheaper sources. World J. Microbiol. Biotechnol.. 2008;24(7):917-925.
- [CrossRef] [Google Scholar]
- Biosurfactant production by Azotobacter chroococcum isolated from the marine environment. Mar. Biotechnol.. 2009;11(5):551-556.
- [CrossRef] [Google Scholar]
- Vaux, D., Cottingham, M. 2001. Method and apparatus for measuring surface configuration. Patent number WO 2007/039729.
- Comparison of methods to detect biosurfactant production by diverse microorganisms. J. Microbiol. Met.. 2004;56(3):339-347.
- [CrossRef] [Google Scholar]