Interfacial characterization of the molecular interactions in mixed monolayers of coumarin and phospholipids
⁎Corresponding author at: Programa de Pós-Graduação em Inovação Terapêutica, Universidade Federal de Pernambuco, 50670-901 Recife, PE, Brazil. csrandrade@pq.cnpq.br (Cesar A.S. Andrade)
-
Received: ,
Accepted: ,
This article was originally published by Elsevier and was migrated to Scientific Scholar after the change of Publisher.
Peer review under responsibility of King Saud University.
Abstract
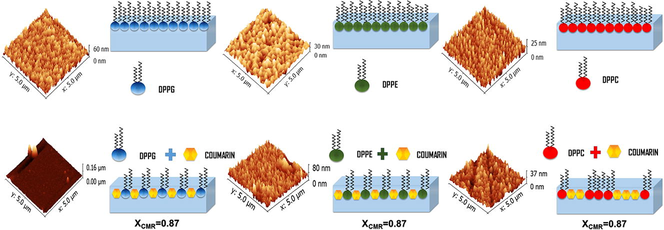
Abstract
Coumarin (CMR) is a small molecule with diverse biological functions as anti-tumor, anti-fungal, anticoagulant and antimicrobial activities. CMR is poorly water soluble molecule with low bioavailability. Lipid-based colloidal carriers play an important role in the delivery of hydrophobic compounds. Langmuir technique is an effective method to evaluate interactions between drugs and lipids. In this study, we used 1,2-dipalmitoyl-sn-glycero-3-phospho-rac-(1-glycerol) (DPPG), 1,2-dipalmitoyl-sn-glycero-3-phosphoethanolamine (DPPE), and 1,2-dipalmitoyl-sn-glycero-3-phosphatidylcholine (DPPC) lipids. Interfacial characterization was carried out based on surface pressure (π)-area (A) isotherms of pure and mixed films. Topographical analysis was performed using atomic force microscopy. The miscibility of the mixed films was dependent on their composition. The mixed films were stable due to the intermolecular attractive interactions. Our results contribute to the understanding of CMR-lipid interactions aiming to obtain stable colloidal carriers for drug delivery.
Keywords
Langmuir films
Langmuir-Blodgett
Coumarin
Phospholipids
Atomic force microscopy
1 Introduction
Plant-derived compounds are known for their antimicrobial activity, treatment, and prevention of infections (Borges et al., 2005; Helander et al., 1998; de Souza et al., 2005). Bioactive compounds, such as benzopyrone class, can be obtained from natural sources as essentials oils extracted from plants (Gill and Holley, 2004; Friedman et al., 2003).
The development of new therapeutic drugs is driven to maximize therapeutic effects and minimize side effects. The production of new active pharmaceutical ingredients is expensive, slow and often uncertain. The natural products played critical roles in modern drug development (Pattni et al., 2015; Fylaktakidou et al., 2004). Coumarin (CMR) and its derivatives are widely distributed throughout nature and exhibit diverse therapeutic applications such as central nervous system stimulants, anti-HIV therapy, anti-coagulant, antitumor, antibacterial and antifungal activities (Musicki et al., 2000; Kostova et al., 2006; Fylaktakidou et al., 2004; Musa et al., 2008). CMR (1,2-benzopyrone) is considered unsuitable for therapeutic use due to its high toxicity, low solubility and limited stability (Hoult and Paya, 1996; Usui, 2006).
Lipid-based colloidal nanostructures are used as carriers for hydrophobic active substances with prospective benefits in biomedicine, cosmetics, and food industry (Rosler et al., 2012; Foldvari et al., 1990; Mozafari et al., 2008; Taylor et al., 2005). In addition, lipid-based colloidal carriers can be targeted to pathological tissues while reducing side effects and dose frequency.
Supported phospholipid monolayers have practical applications such as biomolecular separation (Harlan et al., 1995; Zaitsev et al., 1995), biosensors (Yasuzawa et al., 2000), drug delivery (Torchilin, 2012), and bio-functionalization (Findlay and Barton, 1978). Phospholipids are one of the essential components of the plasma membrane and lipid-based colloidal nanostructures.
The phospholipids used in this study were chosen due to their different size, charge and molecular shapes. Phosphatidylethanolamine (PE) and phosphatidylcholine (PC) are the most important neutral phospholipids found in living organisms (Dowhan, 1997). The abundance of PE is highly variable among organisms and cell types. PC lipids are predominantly found in animal cell membranes (Uran et al., 2001). Phosphatidylglycerol (PG) is a lipid mainly responsible for maintaining membrane lipid surface charge density and permeability to ions (Wada and Murata, 2007).
In this study, we have carried out Langmuir isotherm measurements to analyze the molecular interactions in mixed monolayers composed of phospholipids and CMR. The excess area (
2 Materials and methods
2.1 Materials
Coumarin (99% purity), 1,2-dipalmitoyl-sn-glycero-3-phospho-rac-(1-glycerol), 1,2-dipalmitoyl-sn-glycero-3-phosphoethanolamine and 1,2-dipalmitoyl-sn-glycero-3-phosphatidylcholine (all phospholipids with purity > 99%) were purchased from Sigma–Aldrich (Saint Louis, USA). Ultra-pure water (18.2 MΩ.cm−1) was obtained from a Synergy Milli-Q system (Billerica, USA).
2.2 π-A and ΔV-A isotherms
CMR and lipids solutions were prepared in chloroform at a concentration of 1 mg.mL-1. Subsequently, different solutions of CMR/lipids at different molar ratios (XCMR = 0, 0.250, 0.375, 0.500, 0.675, 0.750, 0.875 and 1) were obtained. Then, 10 µL of these fractions were deposited uniformly on a citrate (citric acid)-phosphate (sodium phosphate) buffer (pH 7.4 and ionic strength 10−3 M) subphase (v = 20 mL) using a Hamilton micropipette. The experiments were performed under symmetrical compression at a constant speed of 15 mm.min−1 (temperature 23 °C ± 0.2 °C), after waiting 15 min to ensure evaporation of the solvent. Isotherms were conducted on a Kibron Langmuir trough (Microtrough XS, Helsinki, Finland). The surface pressure was measured with accuracy of ±0.01 mN.m−1. Surface potential was measured by vibrational plate method (Microspot, Kibron, Helsinki, Finland) with an accuracy of ±0.1 mV. π-A and ΔV-A isotherms were repeated in triplicate with a standard deviation of ∼5%, using at least three different solutions.
2.3 Morphological analysis of LB films
The pure and mixed monolayers were compressed and maintained at target pressure of π = 20 mN.m−1. Subsequently, Langmuir films were transferred onto freshly cleaved mica, dipping the substrate at a constant rate of 2 mm.min−1 in order to control the formation of single monolayers. All measurements were performed with a SPM 9700 atomic force microscopy (Shimadzu instruments Co. ltd, Japan) in a non-contact mode. Cantilevers with a silicon AFM probe (Nanoworld, Japan, resonant frequency = 300 kHz, spring constant = 42 N.m−1) were used. The images (512 points per line) were collected with a scan rate of 1.0 Hz in a scan area of 5.0 × 5.0 µm on at least two different samples on four different areas on each sample In addition, images were obtained and analyzed using AFM Gwyddion software (Necas, 2008).
3 Results and discussion
3.1 Compression isotherms
π-A isotherms of the pure and mixed monolayers versus molecular area are shown in Fig. 1. CMR/DPPG isotherms showed a similar trend to that of pure DPPG lipid (Fig. 1a). Similar phase transitions were observed for all CMR/DPPG mixtures. DPPG and DPPC showed collapse pressures (ΔΠcoll) at 52 mN.m−1 and 60 mN.m−1, respectively. A transition (π ≅ 3 mN.m−1) from liquid expanded to condensed phase was found for pure DPPE monolayer with a Δ. coll at ≅55 mN.m−1. The values obtained for Δ. coll of pure lipids are in agreement with the literature (Vollhardt et al., 2000; Nowotarska et al., 2014).
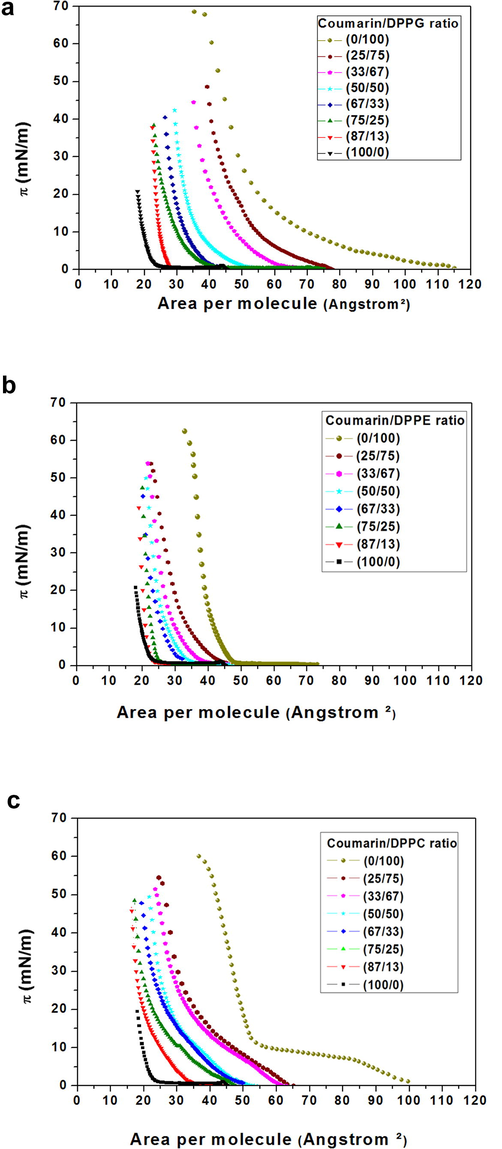
- π-A isotherms of mixed monolayers CMR/DPPG (a), CMR/DPPE (b) and (c) CMR/DPPC at different concentrations.
ΔΠcoll is strongly dependent on the composition of the mixed monolayers and decreases proportionally to CMR molar ratio. From the data presented in Fig. 1a and b it was noticed a steeper rise at xCMR = 0.87 for DPPG and 0.75 for DPPE due to CMR incorporated on these monolayers resulting in a more organized state (Takao et al., 1995).
The interfacial behavior of the isotherms was evaluated considering the difference in the polar region since the studied lipids have identical hydrophobic moieties. DPPE and DPPC are zwitterionic lipids (Fig. 2). DPPG is composed of an anionic polar head (Fig. 2). The polar groups influence the miscibility between the studied molecules resulting in different molecular arrangements (Bouffioux et al., 2007) and lipid packing (Hazell et al., 2016). As expected, DPPG and DPPC mixed films resulted in expanded monolayers (Fig. 1a and c). Pan et al. (2012) demonstrated that DPPG headgroup areas are larger than phosphatidylcholine counterparts as a result of the repulsive electrostatic interactions between charged PG headgroups. DPPC hydrophobic sections are kept farther apart minimizing the lateral cohesive interactions (Myers, 1999). Conversely, DPPE mixed films result in solid-phase monolayers (Fig. 1b).
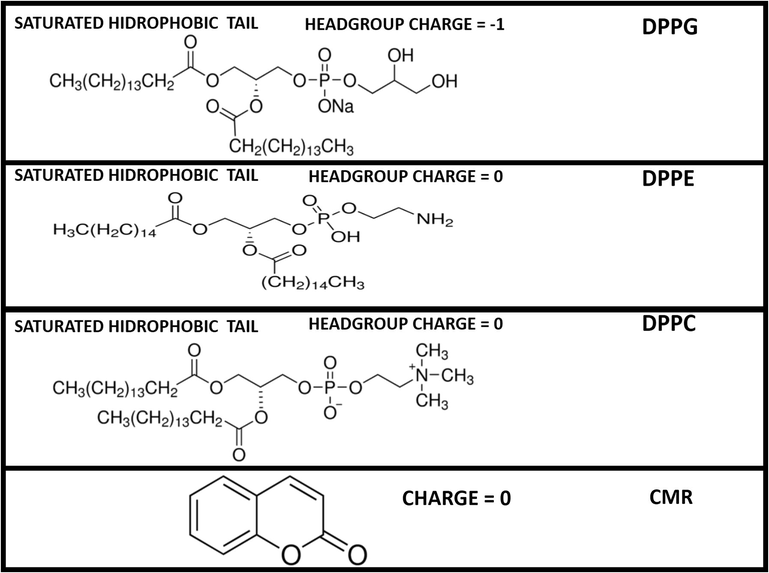
- Phospholipids and coumarin molecular structures. DPPC and DPPC have a cylindrical molecular shape and DPPE has a cone-shaped geometry.
3.2 Surface potential measurements
The surface potential as function molecular area (ΔV-A) is shown in Fig. 3a. ΔV values obtained for all studied phospholipids were similar to previous reports (Andrade et al., 2006; Nowotarska et al., 2014). A gradual decrease of the ΔV (460 mV to 275 mV) as a function of CMR molar fraction is observed for DPPG. ΔV is susceptible to the orientation of both polar and nonpolar groups.
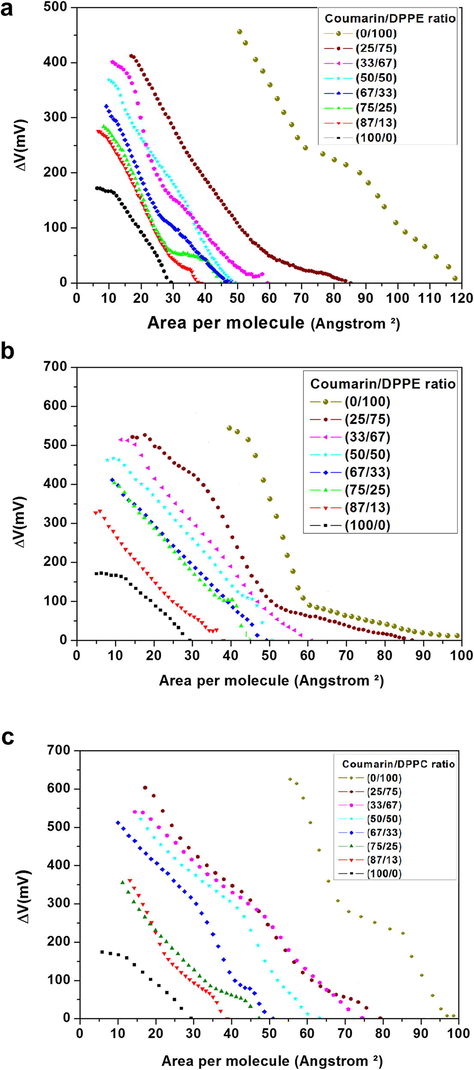
- ΔV-A of CMR/DPPG (a), CMR/DPPE (b) and CMR/DPPC (c) at different concentrations.
A linear dependence of the ΔV for DPPE and DPPC as a function of CMR concentration is observed in Fig. 3b and c, respectively. A decreased of ΔV was obtained for high CMR concentration. In addition, DPPE [DPPC] floating monolayers result in ΔV ∼ 604 [605] mV and for XCMR = 0.87 about of 334 [351] mV. A reduction of the surface potential can be explained through hydrogen bond occurring between CMR and zwitterionic lipid molecule, i.e., between CMR (C=O) acting as hydrogen receptor group and protonated lipid headgroup (e.g. NH3+ found in the DPPE molecules) (Boggs, 1987). DPPG have the ability to form hydrogen bonds between the glycerol moiety and phosphate oxygen of neighboring phosphatidylglycerol lipids (Zaraiskaya and Jeffrey, 2005). DPPE can form inter- and intramolecular hydrogen bonds. Strong intermolecular interactions result in an increase in the liquid-crystalline phase transition temperature, affecting the stability and membrane permeability (Leekumjorn and Sum, 2006). The amine group (hydrogen-donor) of the DPPE can interact strongly with the phosphate/carbonyl groups or water (hydrogen-acceptor). In addition, choline shows a hydrophobic hydration around the CH3 groups, and for amine occurs a competition of hydrogen bonds with water and oxygen atoms in the headgroups (Leekumjorn and Sum, 2006).
CMR interacts with the monolayers tail since it has a lipophilic structure with an octanol-water partition coefficient (log Pow) of = 2.54 (Rabtti, 2012). The binding of molecules to phospholipid tails results in changes of the apparent dipole moment (Hidalgo et al., 2004). The apparent dipole moment is defined by the following formula:
From the data obtained from Eq. 1 we could observe that the relationship of the mixed apparent perpendicular dipole moment (μ) to perpendicular moment of pure phospholipid monolayers (μ0) shifts according to the monolayer compression (Geraldo et al., 2013) (Fig. 4). The μ/μ0 relationship was calculated considering the molecular area at π = 20mN/m (highest ΔΠcoll for pure CMR film). The orientation of the intrinsic molecular dipole (μ) of the molecules forming the film and the organizational structure of water molecules in the subphase is known to play a fundamental role. Of note, μ is considered to be the vector sum of dipole moments arising from the hydrated polar group and hydrocarbon chain (μ = μpolar + μhydrocarbon chain). In addition, DPPG, DPPC and DPPE have similar hydrophobic tail. Thus, it was reasonable to assume that the apolar group contribution to the overall ΔV was the same. The differences in ΔV-A curve profiles were due to their different μpolar contributions.
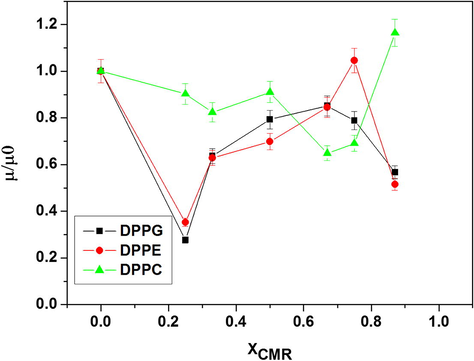
- Apparent dipole moment of pure phospholipid monolayers at π = 20mN/m.
3.3 Thermodynamic analysis
The thermodynamic analysis was performed based on mean molecular area (mma), excess area (ΔAE), excess Gibbs free energy (ΔGe) and excess Gibbs free energy of mixing (ΔGmix) at π = 5, 10, 15, and 20 mN/m. The deviations from linearity of the mma indicate the miscibility of the components according to additivity rule (Szczes et al., 2012) (Fig. 3). ΔAE can be calculated by
The molecular area as a function of the molar ratios of CMR/DPPG is shown in Fig. 5a. It could be seen a negative deviation from mma (XCMR = 0.33–0.67) due to an increase in the molecular attractive interactions. CMR/DPPG films were considered partially miscible and non-ideal floating monolayers since linearity was not observed. The results for CMR/DPPE mixed films exhibited different breaking points at XCMR= 0.25 and 0.67 (Fig. 5b). ΔAE shows an improvement in the packing efficiency or even geometrical accommodations occurred at lower XCMR (Fig. 6) (Andrade et al., 2006; Chou and Chang, 2000).
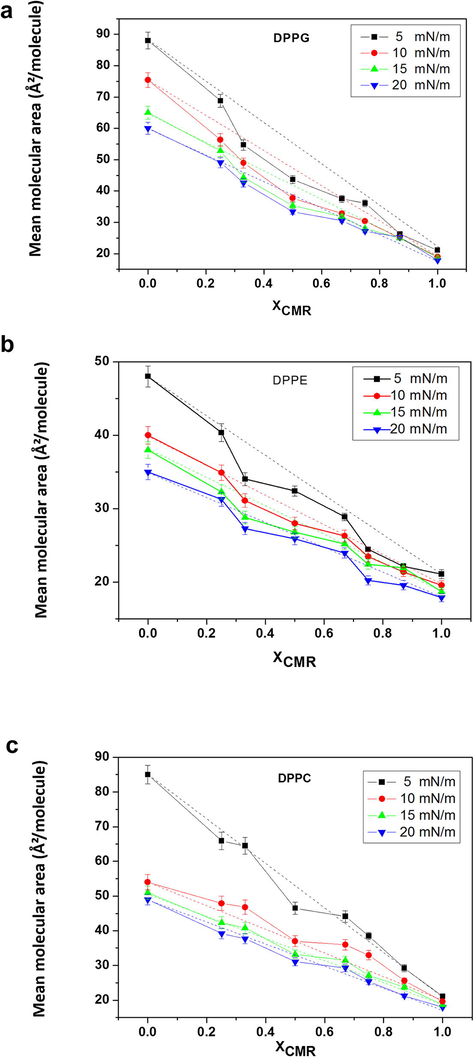
- Mean area per molecule as a function of mole fraction of coumarin in the mixed monolayers at a fixed surface pressure: CMR/DPPG (a), CMR/DPPE (b) and CMR/DPPC (c) at different concentrations (full line: real mean molecular area; dotted line: ideal mean molecular area).
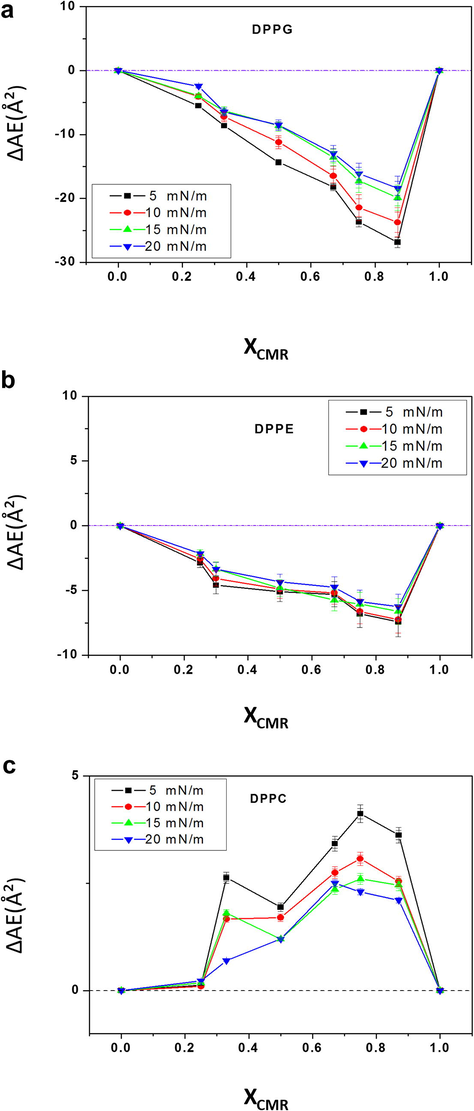
- Excess molecular area of the mixed monolayers of CMR/DPPG (a), CMR/DPPE (b) and CMR/DPPC (c) at different concentrations.
A positive deviation from ΔAE was observed for CMR/DPPC mixed films as a result of a more immiscible behavior (Figs. 5c and 6c). The extent of deviation was dependent on the surface pressure since at higher pressures the molecules become more compact and the effects of intermolecular interactions in the packaging become less evident (Chou and Chang, 2000). The repulsive behavior indicates a phase separation favoring to molecules clustering (Mishra et al., 2012). Similar results have been obtained with other coumarins (Sarpietro et al., 2011; Chakraborty et al., 2012).
In order to analyze the thermodynamic characteristics, we calculated ΔGe and ΔGmix (Baldyga and Dluhy, 1998; Dynarowicz-Latka et al., 2001; Maget-Dana, 1999) as follow:
ΔGe and ΔGmix for CMR/DPPG mixed films indicate a negative deviation from XCMR ≥ 0.25 to XCMR = 1.0 (Figs. 7a and 8a). ΔGe and ΔGmix negative values indicate that CMR/DPPE monolayers are thermodynamically stable (Fig. 7b). ΔGe and ΔGmix values were positive for DPPC mixed monolayers indicating immiscibility (Figs. 7c and 8c). The distance between the molecules is dependent on the phospholipid head group that contributes to the repulsive interactions (Myers, 1999; Boggs, 1987).
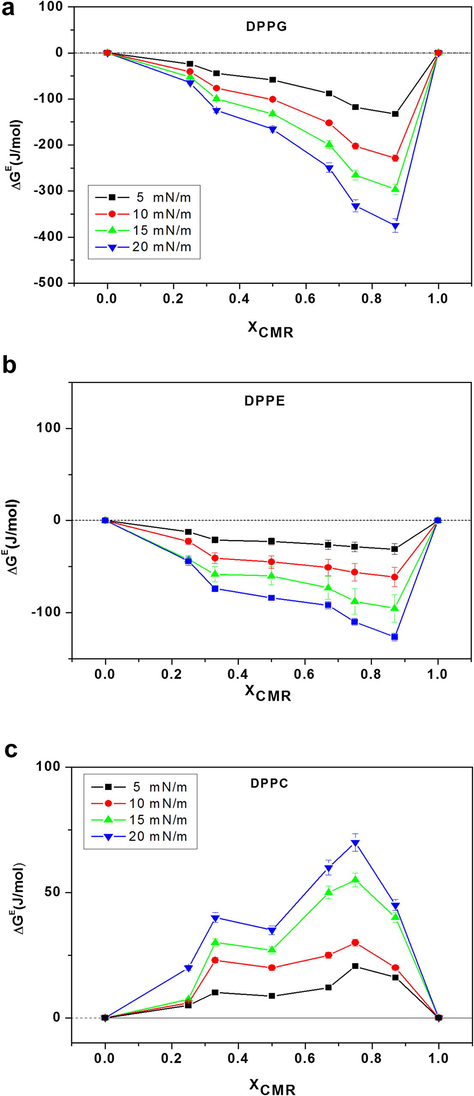
- ΔGe of CMR/DPPG (a), CMR/DPPE (b) and CMR/DPPC (c) at different concentrations.
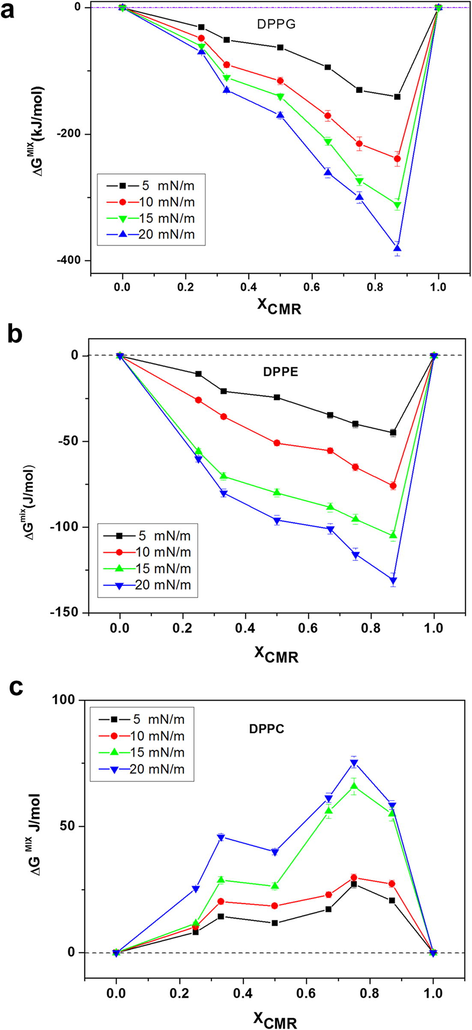
- ΔGmix of CMR/DPPG (a), CMR/DPPE (b) and CMR/DPPC (c) at different concentrations.
3.4 AFM analysis
Fig. 9 shows AFM images of the pure phospholipids monolayers transferred at π = 20 mN/m. Pure phospholipids show a uniform pattern with the root mean square (rms) equivalent to 0.219 nm, 0.060 nm and 0.054 nm for DPPG, DPPE and DPPC, respectively (Fig. 9). We obtained rms ∼ 0.045 nm (XCMR = 0.87) equivalent to a smooth monolayer topography (Fig. 10a). On the other hand, CMR/DPPE and CMR/DPPC films correspond to rough surfaces with rms = 0.1754 nm and 0.072 nm, respectively (Fig. 10b and c). The measurements were repeated in triplicate with a standard deviation of ∼5% for rms.
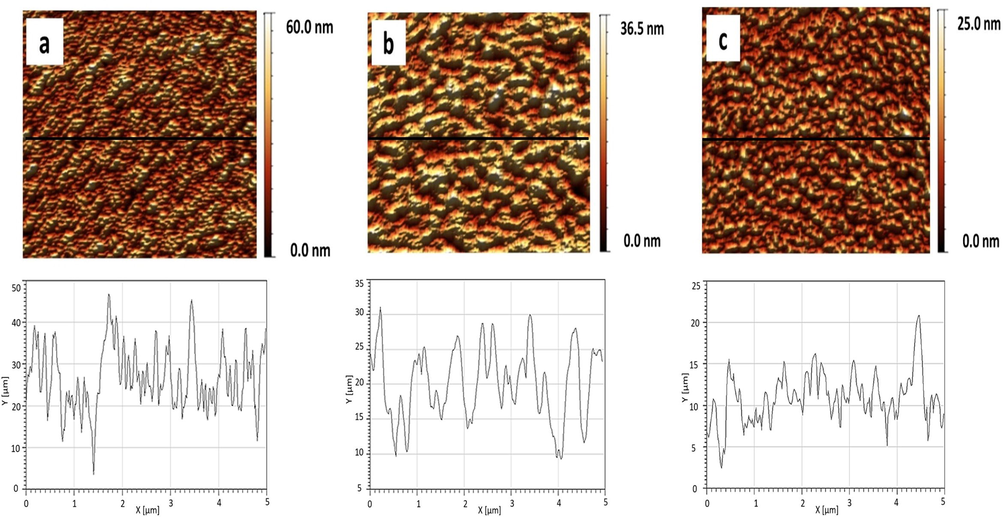
- Topographical images of pure DPPG (a), DPPE (b), and DPPC.
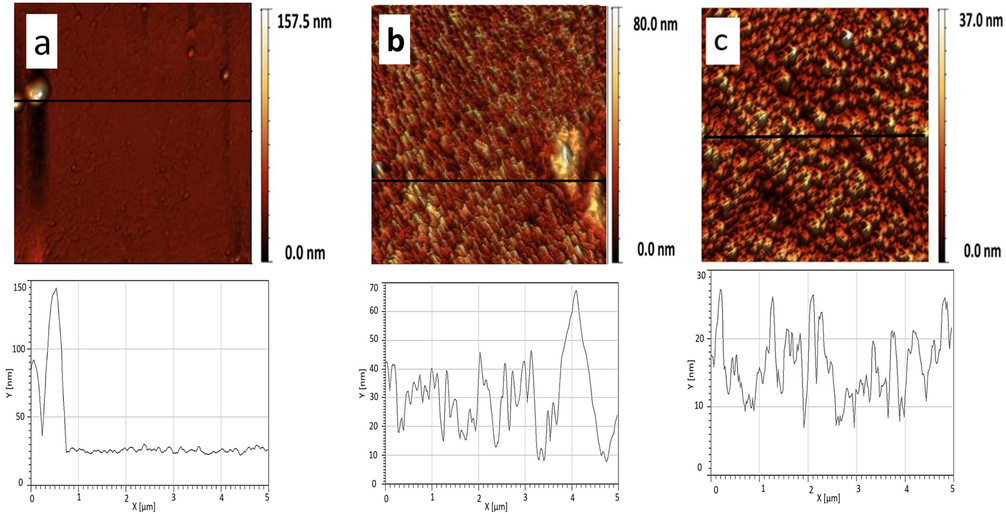
- Topographical images of the mixed monolayers: CMR/DPPG (a), CMR/DPPE (b) and CMR/DPPC (c). The mole fraction of coumarin is equivalent to 0.87.
4 Conclusions
Stability, miscibility and topographical characteristics of pure and mixed monolayers were evaluated by associating Langmuir-Blodgett and AFM techniques. The more effective thermodynamic association was obtained for DPPG/CMR mixed monolayers due to a favorable attractive intermolecular interaction. The differences in the molecular structure of the phospholipids explain the distinct behavior of the interaction between molecules. The coumarin was able to alter the dipole moment, packing of the molecules and aggregate formation.
Acknowledgements
The authors are grateful for the support provided by the FACEPE, CNPq and INCT. Andrade and Oliveira are also gratefully for CNPq financial support (grant 302885/2015-3 and 302930/2015-9, respectively). Rocha would like to thanks FACEPE for a PhD scholarship.
References
- Dielectric properties of Bauhinia monandra and Concanavalin A lectin monolayers, part I. J. Coll. Interf. Sci.. 2005;289(2):371-378.
- [CrossRef] [Google Scholar]
- Thermodynamic characterization of the prevailing molecular interactions in mixed floating monolayers of phospholipids and usnic acid. J. Coll. Interf. Sci.. 2006;298(1):145-153.
- [CrossRef] [Google Scholar]
- On the use of deuterated phospholipids for infrared spectroscopic studies of monomolecular films: a thermodynamic analysis of single and binary component phospholipid monolayers. Chem. Phys. Lipids. 1998;96(1–2):81-97.
- [CrossRef] [Google Scholar]
- Lipid intermolecular hydrogen bonding: influence on structural organization and membrane function. Biochim. Biophys. Acta. 1987;906(3):353-404.
- [Google Scholar]
- Simple coumarins and analogues in medicinal chemistry: occurrence, synthesis and biological activity. Curr. Med. Chem.. 2005;12(8):887-916.
- [Google Scholar]
- Molecular organization of surfactin-phospholipid monolayers: effect of phospholipid chain length and polar head. Biochim. Biophys. Acta. 2007;1768(7):1758-1768.
- [CrossRef] [Google Scholar]
- Formation of nanoscale aggregates of a coumarin derivative in Langmuir-Blodgett film. Appl. Phys. A. 2012;111(4)
- [CrossRef] [Google Scholar]
- Thermodynamic characteristics of mixed DPPC/DHDP monolayers on water and phosphate buffer subphases. Langmuir. 2000;16(7):3385-3390. 10.1021/la990581+
- [Google Scholar]
- Antibacterial activity of coumarins. Zeitschrift Fur Naturforschung C-a J. Biosci.. 2005;60(9–10):693-700.
- [Google Scholar]
- Molecular basis for membrane phospholipid diversity: why are there so many lipids? Ann. Rev. Biochem.. 1997;66:199-232.
- [Google Scholar]
- Modern physicochemical research on Langmuir monolayers. Adv. Colloid Interface Sci. 2001;91(2):221-293.
- [CrossRef] [Google Scholar]
- Phase behavior of synthetic phosphatidylglycerols and binary mixtures with phosphatidylcholines in the presence and absence of calcium ions. Biochemistry. 1978;17(12):2400-2405.
- [Google Scholar]
- Dermal drug delivery by liposome encapsulation - clinical and electron-microscopic studies. J. Microencapsul.. 1990;7(4):479-489.
- [CrossRef] [Google Scholar]
- Antibacterial activities of phenolic benzaldehydes and benzoic acids against Campylobacter jejuni, Escherichia coli, Listeria monocytogenes, and Salmonella enterica. J. Food Prot.. 2003;66(10):1811-1821.
- [Google Scholar]
- Natural and synthetic coumarin derivatives with anti-inflammatory/ antioxidant activities. Curr. Pharm. Des.. 2004;10(30):3813-3833.
- [Google Scholar]
- Langmuir films containing ibuprofen and phospholipids. Chem. Phys. Lett.. 2013;559:99-106.
- [CrossRef] [Google Scholar]
- Mechanisms of bactericidal action of cinnamaldehyde against Listeria monocytogenes and of eugenol against L-monocytogenes and Lactobacillus sakei. Appl. Environ. Microbiol.. 2004;70(10):5750-5755.
- [CrossRef] [Google Scholar]
- Structural characterization of the interaction between a pleckstrin homology domain and phosphatidylinositol 4,5-bisphosphate. Biochemistry. 1995;34(31):9859-9864.
- [Google Scholar]
- Langmuir monolayers composed of single and double tail sulfobetaine lipids. J. Coll. Interf. Sci.. 2016;474:190-198.
- [CrossRef] [Google Scholar]
- Characterization of the action of selected essential oil components on gram-negative bacteria. J. Agric. Food Chem.. 1998;46(9):3590-3595. 10.1021/jf980154m
- [Google Scholar]
- Interaction of two phenothiazine derivatives with phospholipid monolayers. Biophys. Chem.. 2004;109(1):85-104.
- [CrossRef] [Google Scholar]
- Pharmacological and biochemical actions of simple coumarins: natural products with therapeutic potential. Gen. Pharmacol.. 1996;27(4):713-722.
- [Google Scholar]
- Structure-activity relationships of synthetic coumarins as HIV-1 inhibitors. Bioinorg. Chem. Appl.. 2006;68274
- [CrossRef] [Google Scholar]
- Molecular simulation study of structural and dynamic properties of mixed DPPC/DPPE bilayers. Biophys. J.. 2006;90(11):3951-3965.
- [CrossRef] [Google Scholar]
- The monolayer technique: a potent tool for studying the interfacial properties of antimicrobial and membrane-lytic peptides and their interactions with lipid membranes. Biochim. Biophys. Acta. 1999;1462(1–2):109-140.
- [CrossRef] [Google Scholar]
- Investigation of Miscibility and Aggregate Formation in the Mixed Langmuir-Blodgett Films of 2-aminoanthracene by Surface Pressure and Spectroscopic Methods. Mol. Cryst. Liq. Cryst. 2012;557(1)
- [CrossRef] [Google Scholar]
- Nanoliposomes and their applications in food nanotechnology. J. Liposome Res.. 2008;18(4):309-327.
- [CrossRef] [Google Scholar]
- A review of coumarin derivatives in pharmacotherapy of breast cancer. Curr. Med. Chem.. 2008;15(26):2664-2679.
- [Google Scholar]
- Improved antibacterial activities of coumarin antibiotics bearing 5',5'-dialkylnoviose: biological activity of RU79115. Bioorg. Med. Chem. Lett.. 2000;10(15):1695-1699.
- [Google Scholar]
- Surfaces, interfaces, and colloids: principles and applications (2nd ed.). New York: Wiley-VCH; 1999.
- Necas, D., K., P., Anderson, C., Gwyddion, 2008.
- Effect of structure on the interactions between five natural antimicrobial compounds and phospholipids of bacterial cell membrane on model monolayers. Molecules. 2014;19(6):7497-7515.
- [CrossRef] [Google Scholar]
- Molecular structures of fluid phase phosphatidylglycerol bilayers as determined by small angle neutron and X-ray scattering. Biochim. Biophys. Acta. 2012;1818:2135-2148.
- [CrossRef] [Google Scholar]
- New developments in liposomal drug delivery. Chem. Rev.. 2015;115(19):10938-10966.
- [CrossRef] [Google Scholar]
- Rabtti, E.H.M.A., Natic, Maja M., Milojkovic-Opsenica, Dušanka, M., Trifkovic, Jelena Ð, Vuckovic, Ivan M., Vajs, Vlatka E., Tešic, Živoslav Lj, 2012. RP TLC-based lipophilicity assessment of some natural and synthetic coumarins. J. Braz. Chem. Soc. 23, 522–530.
- Advanced drug delivery devices via self-assembly of amphiphilic block copolymers. Adv. Drug Deliv. Rev.. 2012;64:270-279.
- [CrossRef] [Google Scholar]
- Sarpietro, M.G., Giuffrida, M.C., Ottimo, S., M., D., Castelli, F., 2011. Evaluation of the interaction of coumarins with biomembrane models studied by differential scanning calorimetry and Langmuir-Blodgett techniques. J. Nat. Prod. 74(4). doi: 10.1021/np100850u
- Stability of binary model membranes-prediction of the liposome stability by the Langmuir monolayer study. J. Coll. Interf. Sci.. 2012;372:212-216.
- [CrossRef] [Google Scholar]
- Molecular interactions between lipids and some steroids. Langmuir. 1995;11(3):912-916.
- [CrossRef] [Google Scholar]
- Liposomal nanocapsules in food science and agriculture. Crit. Rev. Food Sci. Nutr. 2005;45(7–8):587-605.
- [CrossRef] [Google Scholar]
- Analysis of phospholipid species in human blood using normal-phase liquid chromatography coupled with electrospray ionization ion-trap tandem mass spectrometry. J. Chromatogr. B Biomed. Sci. Appl.. 2001;758(2):265-275.
- [Google Scholar]
- Thermodynamic and textural characterization of DPPG phospholipid monolayers. J. Phys. Chem. B. 2000;104(17):4115-4121.
- [CrossRef] [Google Scholar]
- The essential role of phosphatidylglycerol in photosynthesis. Photosynthesis Res.. 2007;2:205-215.
- [CrossRef] [Google Scholar]
- Preparation of glucose sensors using the Langmuir-Blodgett technique. Sens. Actuators B. 2000;65:241-243.
- [Google Scholar]
- Mixed monolayers of valinomycin and dipalmitoylphosphatidic acid. Colloids Surfaces Colloids Surf. A. 1995;94:75-83.
- [Google Scholar]
- Molecular dynamics simulations and 2H NMR study of the GalCer/DPPG lipid bilayer. Biophys. J.. 2005;6:4017-4031.
- [Google Scholar]