Synthesis, spectral, thermal and antibacterial studies of Cd(II), Mn(II) and Fe(III) complexes containing trithiocarbonate 1,3,4-thiadiazole moiety
*Corresponding author. Address: Department of Chemistry, College of Science for Women, University of Baghdad, Jadiriyah, P.O. Box 47162, Iraq. Tel.: +964 7902789368 carolin.sh86@yahoo.com (Carolin Shakir)
-
Received: ,
Accepted: ,
This article was originally published by Elsevier and was migrated to Scientific Scholar after the change of Publisher.
Available online 1 December 2012
Peer review under responsibility of King Saud University.
Abstract
A new metal complex of Cd(II), Mn(II) and Fe(III) derived from potassium 2-carbomethoxy amino-5-trithiocarbonate 1,3,4-thiadiazole (CATT) and potassium 2-N(4-N,N-dimethylaminobenzyliden)-4-trithiocarbonate1,3,4-thiadiazole (DBTT), has been synthesized. Structural features of these complexes were obtained in the solid state by several techniques using flame atomic absorption, elemental analyses C, H, N and S, FT-IR, UV–vis spectra, thermal analyses TGA, conductivity and magnetic susceptibility measurements. Spectral data suggest an octahedral structure around the metal ion except cadmium (II) complexes that have tetrahedral geometry. The antibacterial activity of ligands and their metal complexes in vitro against the microorganisms: Pseudomonas aeruginosa (as gram negative strain bacteria) and Staphylococcus aureus (as gram positive strain bacteria) was examined using two different concentrations (10 and 5 mM) in nutrient agar media and some complexes showed noticeable activity against the tested microorganisms comparaing them to ampicillin as the standard drug.
Keywords
Trithiocarbonate
Thiadiazole
Techniques
In vitro
Microorganisms
1 Introduction
The thiadiazole ring and its derivatives posses good coordination behavior, since they have a sulfur atom and two nitrogen atoms in addition to the substituent having a donating group in the structure (March and Smith, 2007), therefore the study of its metallic complexes is of structural importance in addition to many important applications such as fungicidal and leshmanicidal activities (Łukaszuket al., 2007; Foroumadi et al., 2005). Trithiocarbonate complexes have received attention because of the dual nature of the metal-CS3 moiety as an electrophilic and nucleophilic reagent, which makes them versatile intermediates for the synthesis of other thio species by their participation in (i) alkylation at the exocyclic sulfur atom, (ii) displacement of weakly bound ligands from metal complexes, (iii) reaction with unsaturated species to give binuclear complexes, (iv) cycloaddition of activated alkynes, (v) sulfur extraction to give CS2 complexes, or (vi) sulfur addition to give
In the present work, new derivatives of the trithiocarbonate with acetate and azomethine moieties in the structure of the thiadiazole ring were synthesized, and it Cd(II), Mn(II) and Fe(III) complexes were prepared to investigate the coordination behavior of these new compound and their antibacterial activity.
2 Experimental
2.1 Instrumentation
All the chemicals were of analytical analar grade. Metal salts used in this study are cadmium chloride dihydrate CdCl2·2H2O from (BDH), magnesium acetate tetrahydrate Mn(OAc)2·4H2O from (Fluka) and ferric chloride tetrahydrate FeCl3·4H2O from (Fluka). The metal analyses were performed by a Perkin Elmer 5000 Atomic Absorption Spectrophotometer. Elemental analyses (C, H, N and S) of compounds were carried out with EM-034·mth. The electronic spectra were recorded on a Shimadzu (UV–vis 1600A) Ultra Violate Spectrophotometer using a quartz cell at a wave length range of 200–1100 nm. FT-IR spectra were recorded on a Shimadzo 8400 Fourier Transform Spectrophotometer by using a CsI disc in the wave number range of 4000–200 cm−1. Thermal analysis TGA was performed with 4000 Perkin–Elmer. Magnetic susceptibility measurements were determined using the Magnetic Susceptibility Balance of the Johnson matting catalytic system division, England at room temperature. The molar conductance was measured in DMSO as a solvent at room temperature using Coring Conductivity Meter 220. Melting point apparatus of Gallencamp M.F.B-600.01 was used.
2.2 Synthesis of ligands (CATT & DBTT)
2.2.1 Synthesis of potassium 2-carbomethoxy amino-5-trithiocarbonate mercapato 1,3,4-thiadiazole (CATT)
This ligand was prepared by the reaction of methyl chloroformate with 2-amino-5-mercapto1,3,4-thiadiazole in the presence of pyridine, methyl chloroformate (1.16 ml) was added drop wise to a solution of (2 g, 1.5 mmol) 2-amino-5-mercapto-1,3,4-thiadiazole in (5.42 ml) pyridine with continuous stringing for 1 h, the mixture was diluted with (24.63 ml) cold distilled water and dilute HCl at pH 3. A pale precipitate was formed collected by filtration washed excessively with cold distilled water and dried in an oven at 70°C. 2 g, 0.01 mmol of the previous mixture was dissolved in 20 ml of absolute ethanol in the presence of 1.5 ml CS2 and 0.58 g, 0.01 mol of KOH was added as alkali media. The mixture was refluxed for 3 h, then the solvent was evaporated and the precipitate (Scheme 1) was filtrated, then recrystallized from ethanol and distilled water. The physical properties are shown in (Table 1).
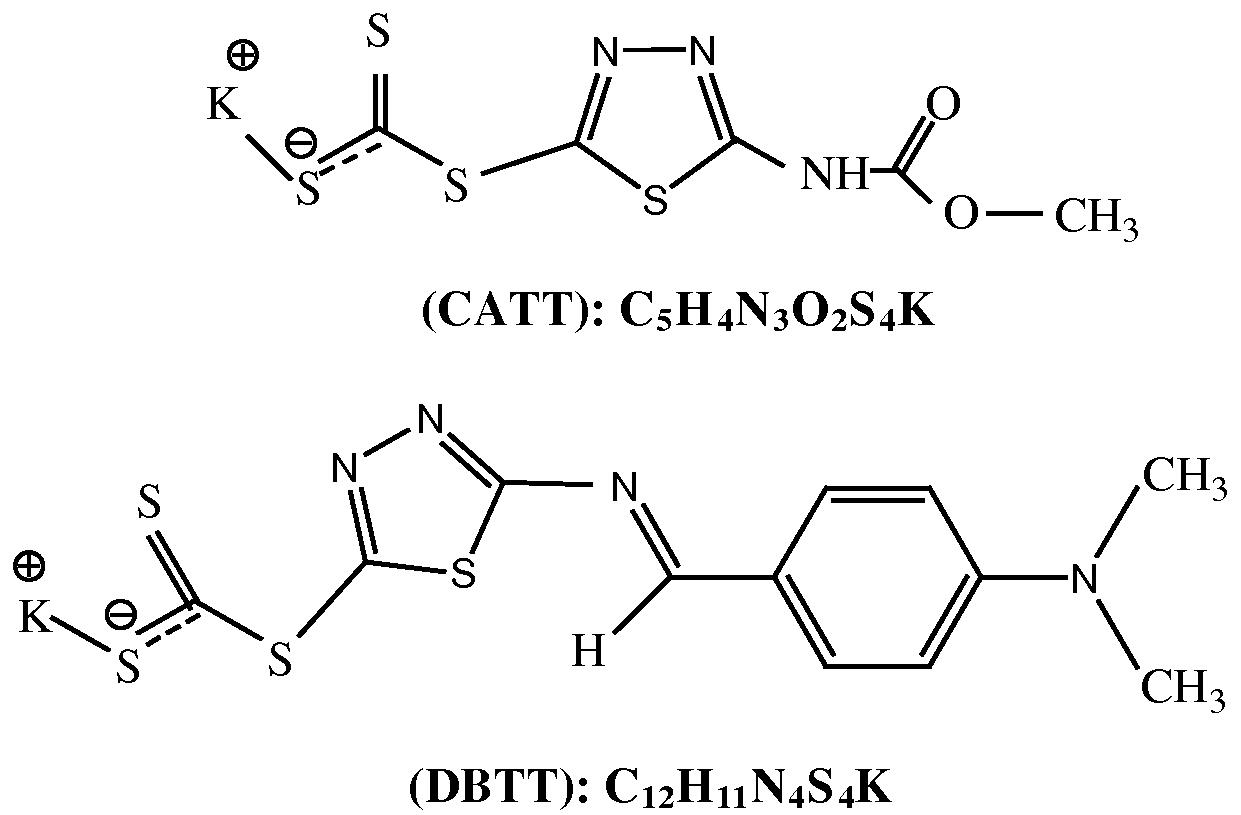
- Structures of the new ligands CATT & DBTT.
Comp. | Color | M.P. (°C) | Yield (%) | M.Wt (g mol−1) | Elemental analyses found (Calc.) | Metal percentage | ||||
---|---|---|---|---|---|---|---|---|---|---|
C | H | N | S | Found | Calc. | |||||
(CATT) | Yellow | 210 | 62.2 | 305.09 | 19.45 | 1.20 | 13.99 | 41.69 | – | – |
(19.66) | (1.31) | (13.76) | (41.95) | |||||||
Cd(CATT)2 | Off white | 240d | 54.3 | 782.41 | 24.03 | 3.11 | 10.30 | 32.35 | 14.05 | 14.36 |
(24.54) | (3.32) | (10.73) | (32.72) | |||||||
Mn(CATT)2 | Brown | 190 | 71.9 | 668.93 | 21.27 | 2.48 | 12.45 | 38.05 | 7.90 | 8.21 |
(21.52) | (2.69) | (12.55) | (38.27) | |||||||
Fe2(CATT)4 | Brown | 120d | 55.7 | 1282.69 | 18.38 | 1.67 | 13.32 | 39.44 | 8.54 | 8.70 |
(18.71) | (1.56) | (13.09) | (39.91) | |||||||
(DBTT) | Dark orange | 150 | 70.0 | 378.09 | 38.25 | 2.98 | 14.55 | 33.53 | – | – |
(38.08) | (2.91) | (14.81) | (33.85) | |||||||
Cd(DBTT) | Light orange | 270d | 66.5 | 670.91 | 35.25 | 5.75 | 8.18 | 19.47 | 16.32 | 16.75 |
(35.77) | (5.21) | (8.34) | (19.07) | |||||||
Mn(DBTT) | Brown | 235d | 70.02 | 506.93 | 33.27 | 3.68 | 11.35 | 25.66 | 10.65 | 10.83 |
(33.14) | (3.94) | (11.04) | (25.25) | |||||||
Fe(DBTT) | Dark brown | 260d | 68.8 | 575.84 | 33.92 | 3.77 | 9.92 | 21.95 | 9.60 | 9.70 |
(33.34) | (3.99) | (9.72) | (22.22) |
d = decomposition degree.
2.2.2 Synthesis of potassium 2-N(4-N,N-dimethylaminobenzyliden)-4-trithiocarbonate 1,3,4-thiadiazole (DBTT)
A mixture of (2 g, 0.015 mmol) 2-amino-5-mercapto1,3,4-thiadiazole and (3.07 g, 0.015 mmol) of 4,-N,N-dimethylaminobenzyliden in 10 ml of absolute ethanol and one drop of glacial acetic acid with continuous stringing was heated in a water bath at 60–70°C and reflexed for 1 h, then left to cool in an ice-water bath were an orange precipitate was obtained. 2 g, 0.0075 mol of the previous mixture, that is, 2-N(4,4-dimethylamin benzyliden)-5-mercapto1,3,4-thiadiazole in (20 ml) of absolute ethanol was added to 1.5 ml of CS2 and 0.42 g, 0.0075 mol of KOH as alkali media. The mixture was refluxed for 3 h then the solvent was distilled off and the precipitated crystal was filtered and recrystallized from ethanol and distilled water to obtain an orange precipitate (Scheme 1). The physical properties can be shown in (Table 1).
2.3 Synthesis of complexes
New (CATT) (0.610 g, 2 mmol) in all complexes which contain a 1:2 ratio of M:L was dissolved in 10 ml of absolute ethanol, while 0.378 g, 1 mmol of complexes of the (DBTT) ligand which contains a 1:1 ratio of M:L also was dissolved in 10 ml of absolute ethanol followed by the addition (5 ml) of the metal salt drop by drop in ethanol and mixed together. The reaction mixture was allowed to stir magnetically for 1 h at room temperature. The resulting precipitate was filtered and washed with water and recrystallized from hot ethanol, then dried at 50°C for 1 h (Table 1).
2.4 Biological activity
The in vitro biological activity of the investigated ligands and their metal complexes was tested against the bacteria Pseudomonas aeruginosa and Staphylococcus aureus by disc diffusion method using nutrient agar as the medium. Each of the compounds was dissolved in DMSO and solutions of the concentrations (5 and 10 mM) were prepared separately. In a typical procedure, a well was made on an agar medium inoculated with the microorganism. The well was filled with the test solution using a micropipette and the plate was incubated for 24 h at 37°C. During this period, the test solution diffused and the growth of the inoculated microorganism was affected. The inhibition zone was developed, at which the concentration was noted.
3 Results and discussion
3.1 Infrared spectra
The FT-IR spectra analyses gave good information about the mode of coordination of the CATT and DBTT ligands to the metallic ions. The characteristic bands are presented in (Table 2). In the CATT ligand spectrum νNH vibration from the imide group is located at 3210 cm−1 which remained unchanged in all complexes also the band exhibited at 1566 cm−1 indicates the presence of the azomethine group νC⚌N which also remained without change in all the complexes (Bakkar et al., 2010; Maradiya and Patel, 2001), νNCS shows a band at a 1427 cm−1 that does not undergo any shifting in all the complexes (Nakomato, 2009). The frequencies appeared at 1730 and 1083 cm−1 which is attributed to νC⚌O and νCOC groups which remained unchanged in all the complexes for νC⚌O group while they show neglectable change for νCOC (Singh et al., 2012; Nakomato, 2009), another three bands appeared which attributed to the trithiocarbonate SCS2 group located at 1047, 752 and 952 cm−1 which are assigned to the νC⚌S, νC–S and νC—S bands respectively, the νC⚌S and νC—S which undergo shift to lower frequencies in all the complexes except in the Mn(II) complex which exhibits shift to higher frequency in the νC⚌S group only, while the νC–S group undergos shift to higher frequencies in all the complexes and this supports the coordination of metal ions with the ligand (CATT) by these groups, that is, νC–S and νC—S (Vicente et al., 1997, 1995). The coordination of the trithiocarbonate of the (CATT) ligand to the Cd(II), Mn(II) and Fe(III) ions was also proved by the νM–S vibrations appearing in the range of 401–470 cm−1 which was absent in the spectrum of the ligand (Jain and Mishra, 2012). In the DBTT ligand exhibit νC⚌N band of the ring at 1527 cm−1 remained unchanged in all complexes, the νC⚌N band at 1591 cm−1 was observed due to the azomethine group which shows a shift to lower frequencies in all complexes almost between 10 and 31 cm−1 which may be refered to as the coordination of the ligand with metal ions by this group (Mitu et al., 2012), the νNCS band at the 1440–1450 cm−1 region was observed. The frequencies that appeared at 1031, 777 and 943 cm−1 are assigned to νC⚌S, νC–S and νC—S bands respectively, which undergo shift to higher frequencies in all complexes for νC–S, while they undergo shift to lower frequencies in all complexes for νC⚌S and νC—S (Vicente et al., 1997, 1995) and this refers to the coordination of metal ions with (DBTT) by both νC–S and νC—S bonds in addition to the νC⚌N group, another band exhibited at 1371 cm−1 refers to the νAr–N mode which undergoes a slight shift about 2 cm−1 in Cd(II) and Mn(II) complexes. Other new bands appeared which were supported by the appearance of frequencies of ν(M–S), ν(M–N), ν(M–Cl) and ν(M–O) bonds. A band observed around 3360–3417 cm−1 in the spectra of metal complexes, assigned to the νOH which refers to the presence of uncoordinated EtOH or H2O (Nakomato, 2009; Soleimani, 2010).
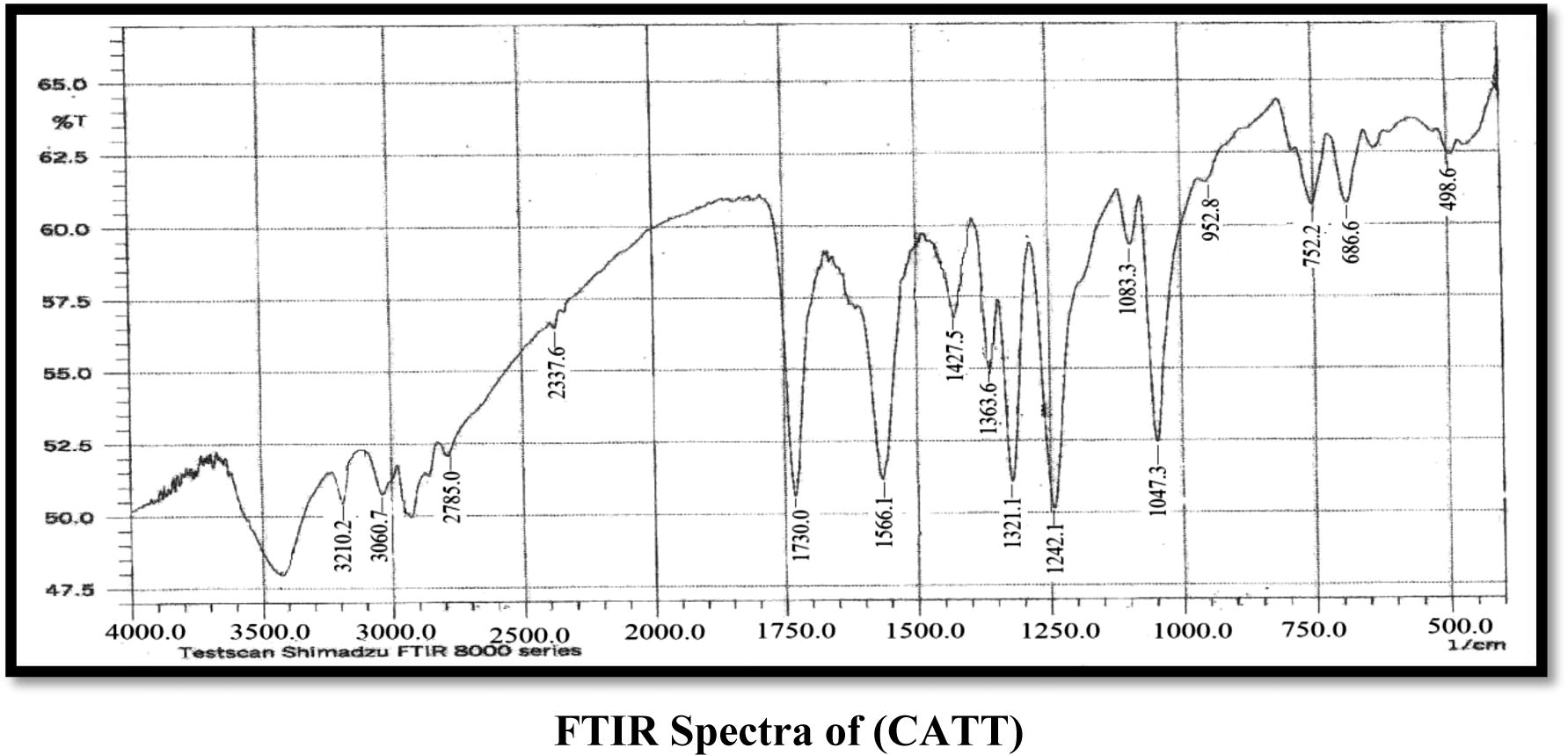
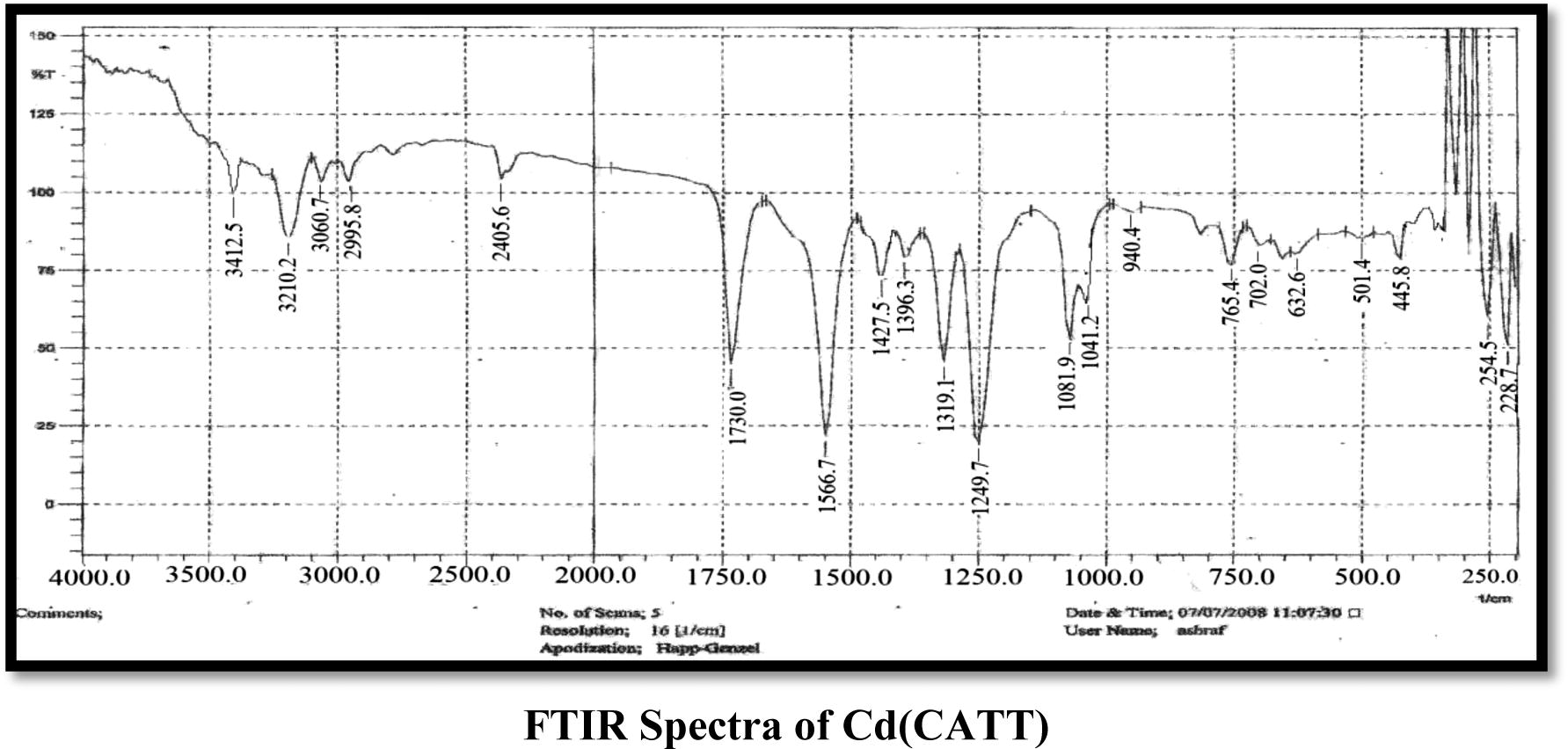
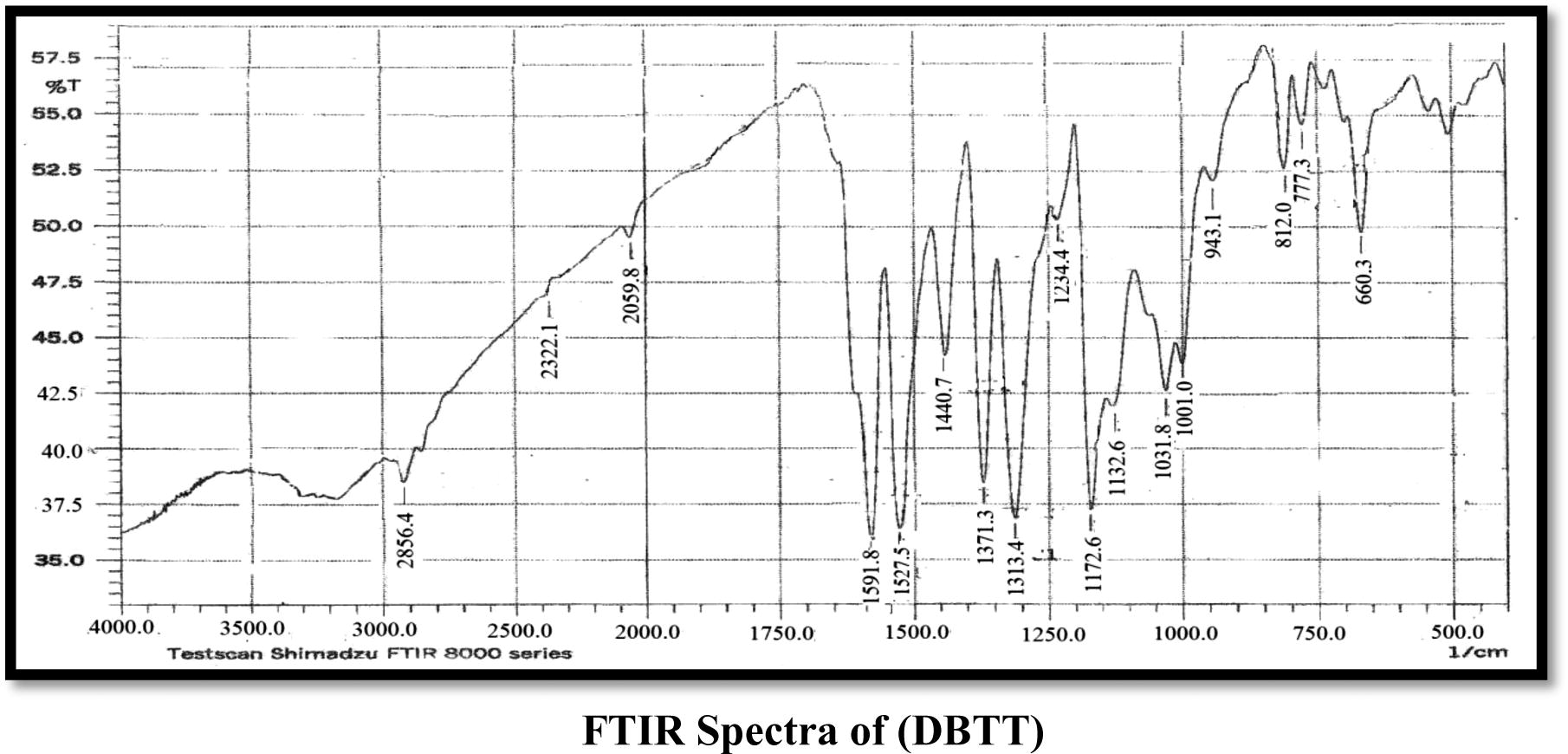
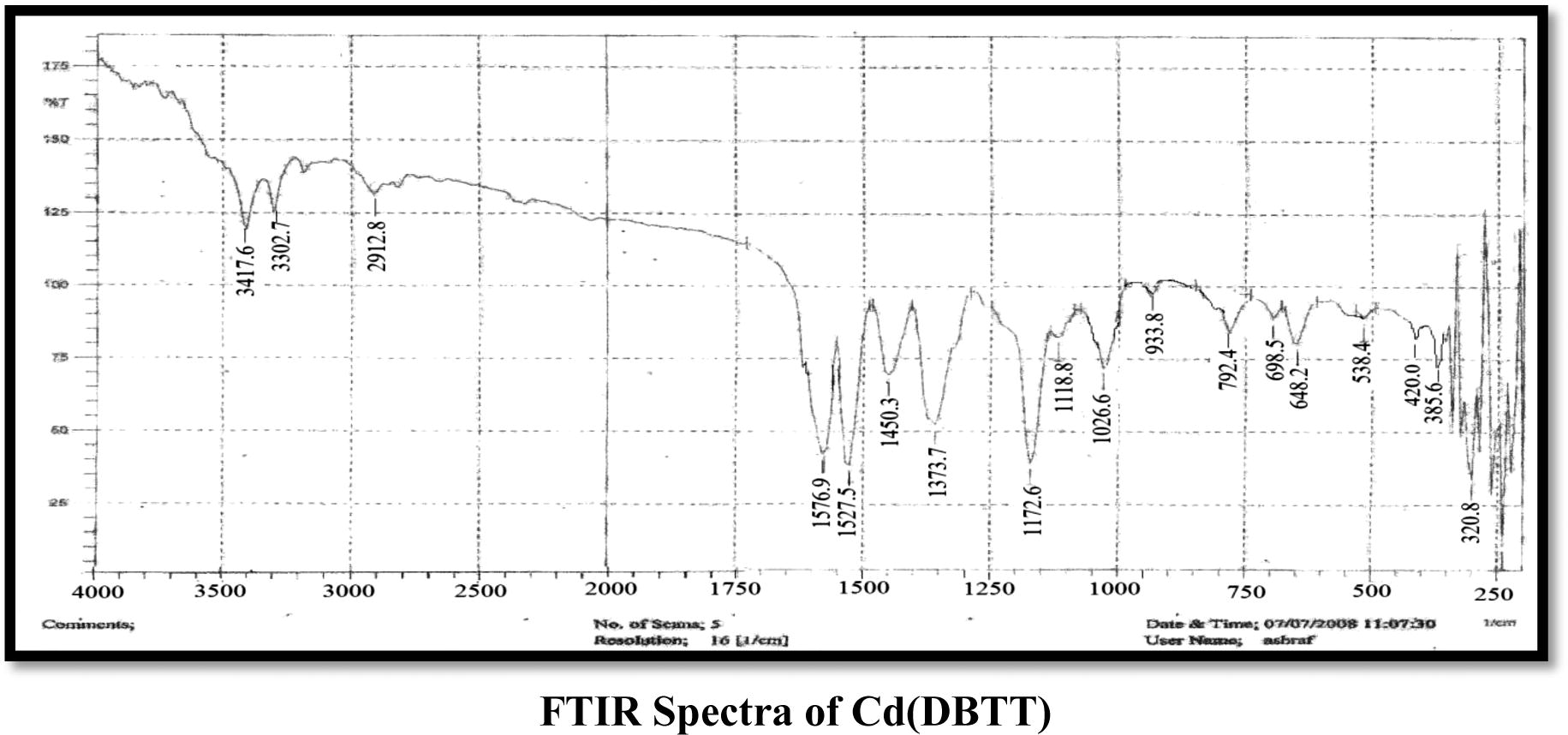
Comp. | νN–H | νC⚌N ring | νC⚌N | νNCS | νC⚌O | νCOC | νAr–N | νC⚌S | νC–S | νC⋯S | νM–N | νM–S | νM–X |
---|---|---|---|---|---|---|---|---|---|---|---|---|---|
(CATT) | 3210 | 1566 | – | 1427 | 1730 | 1083 | – | 1047 | 752 | 952 | – | – | – |
Cd(CATT)2 | 3210 | 1566 | – | 1427 | 1730 | 1081 | – | 1041 | 765 | 940 | – | 445 | – |
Mn(CATT)2 | 3210 | 1566 | – | 1427 | 1730 | 1082 | – | 1056 | 786 | 941 | – | 401 | M–O 478 |
Fe2(CATT)4 | 3210 | 1566 | – | 1427 | 1730 | 1082 | – | 1040 | 763 | 942 | – | 470 | M–Cl 378 |
(DBTT) | – | 1527 | 1591 | 1440 | – | – | 1371 | 1031 | 777 | 943 | – | – | – |
Cd(DBTT) | – | 1527 | 1576 | 1450 | – | – | 1373 | 1026 | 792 | 933 | 538 | 420 | M–Cl 385 |
Mn(DBTT) | – | 1527 | 1560 | 1448 | – | – | 1373 | 1022 | 789 | 932 | 540 | 416 | M–O 478 |
Fe(DBTT) | – | 1527 | 1581 | 1448 | – | – | 1371 | 1026 | 786 | 930 | 535 | 424 | M–Cl 392 M–O 488 |
3.2 Electronic spectra and magnetic moment
The nature of the ligand field around the metal ion has been deduced from the electronic spectra. The electronic spectra of these compounds were recorded as solution in the DMSO solvent.
3.2.1 Cd(II) complexes
The electronic spectra of the Cd(II) complex of the CATT ligand exhibit two transition bands at 23.786 and 28.571 cm−1, while those of the DBTT ligand of the Cd(II) complex show three bands at 22.347, 27.027 and 32.894 cm−1 assigned to charge transfer transition (Solomon and Lever, 2006) (Table 3). Thus, the tetrahedral geometry has been suggested for these complexes. The conductivity measurements in DMSO at room temperature showed both complexes to be non-electrolytic in nature.
Comp. | Absorption bands (cm−1) | Assignment | B° | B/ | β | Dq/B/ | 10Dq | 15B/ | μeff B.M. | Nature of bond | μscm−1 | Suggested geometry |
---|---|---|---|---|---|---|---|---|---|---|---|---|
Cd (CATT)2 | 23.786 28.571 |
ILCT | – | – | – | – | – | – | – | – | 26 | Td |
Mn (CATT)2 | 11.688 | 6A1g→4T1g(G) | 860 | 550.5 | 0.64 | 1.9 | 10.459 | 9.789 | 4.88 | Covalent | 18 | Oh |
18.348 | 6A1g→4T2g(G) | |||||||||||
26.505 | 6A1g→6A1g + 4Eg | |||||||||||
Fe2 (CATT)4 | 11.695 | 6A1g→4T1g | 1015 | 771.4 | 0.76 | 1.3 | 10.028 | 9.748 | 5.16 | Ionic | 15 | Oh |
18.518 | 6A1g→4T2g | |||||||||||
26.315 | 6A1g→6A1g + 4Eg | |||||||||||
Cd (DBTT) | 22.347 | ILCT | – | – | – | – | – | – | – | – | 18 | Td |
27.027 | ||||||||||||
32.894 | ||||||||||||
Mn (DBTT) | 12.658 | 6A1g→4T1g(G) | 860 | 452.6 | 0.52 | 2.5 | 11.315 | 8.557 | 4.90 | Covalent | 26 | Oh |
18.765 | 6A1g→4T2g(G) | |||||||||||
27.766 | 6A1g→6A1g + 4Eg | |||||||||||
Fe (DBTT) | 11.960 | 6A1g→4T1g | 1015 | 829.2 | 0.81 | 1.35 | 11.194 | 10.685 | 4.91 | Ionic | 27 | Oh |
19.615 | 6A1g→4T2g | |||||||||||
26.950 | 6A1g→6A1g + 4Eg |
ILCT: Internal ligand charge transfer.
3.2.2 Mn(II) complexes
In the present work, the brown color of the Mn(II) complexes shows three bands at 11.688–12.658, 18.348–18.765 and 26.505–27.766 cm−1 assigned to 6A1g→4T1g(G), 6A1g→4T2g(G) and 6A1g→6A1g + 4Eg transitions which refer to octahedral geometry (Ghanim, 2012; Solomon and Lever, 2006) (Table 3). The μeff value ranged between 4.88 and 4.90 B.M. and this supports the suggested geometry of this complex (Singh et al., 2012), respectively and the conductivity measurement shows that the complexes is non-electrolytic in nature.
3.2.3 Fe(III) complexes
The absorption spectrum of the Fe(III) complexes of CATT and DBTT shows bands in the range of 11.695–11.960, 18.518–19.615 and 26.315–26.950 cm−1, which may be assigned to 6A1g→4T1g (ν1), 6A1g→4T2g (ν2) and 6A1g→6A1g + 4Eg (ν3) transitions, respectively (Solomon and Lever, 2006; Deshmukh et al., 2010). The magnetic moment is 4.91–5.16 B.M., thus octahedral geometry has been suggested for these complexes (Kumar et al., 2012). The value of various ligand field parameters, that is, 10Dq, B/, β and 15B/ were calculated using Tanabe-Sugano diagram for the d3 system (Table 3). The conductance measurements indicate the non-conducting behaviors of these complexes.
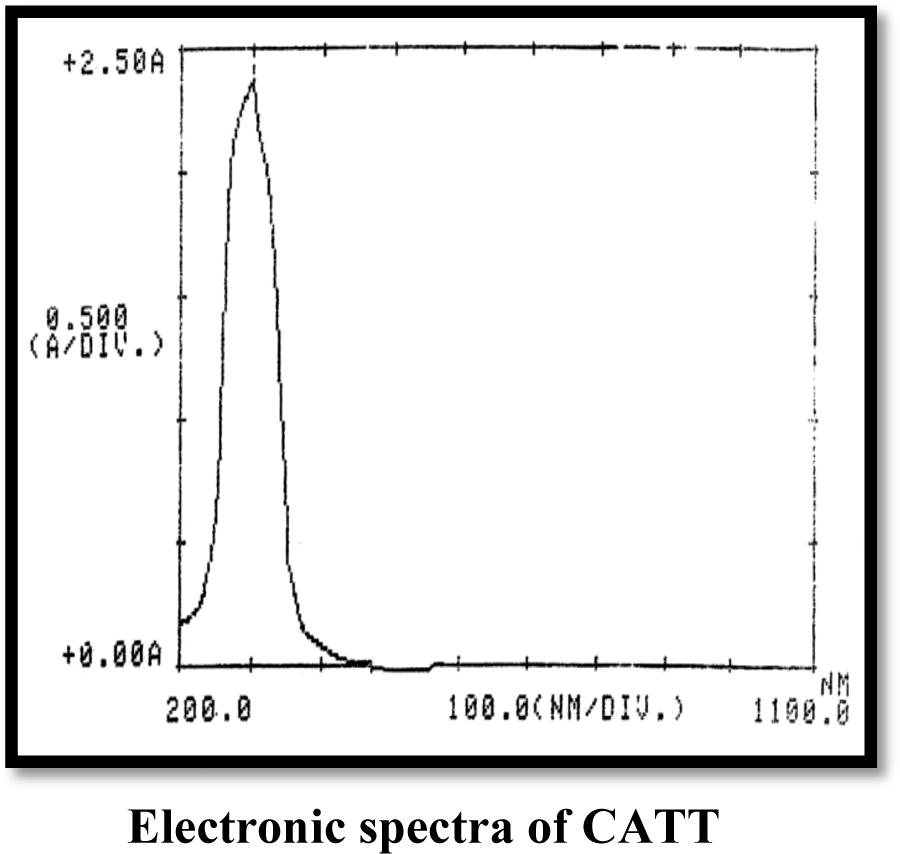
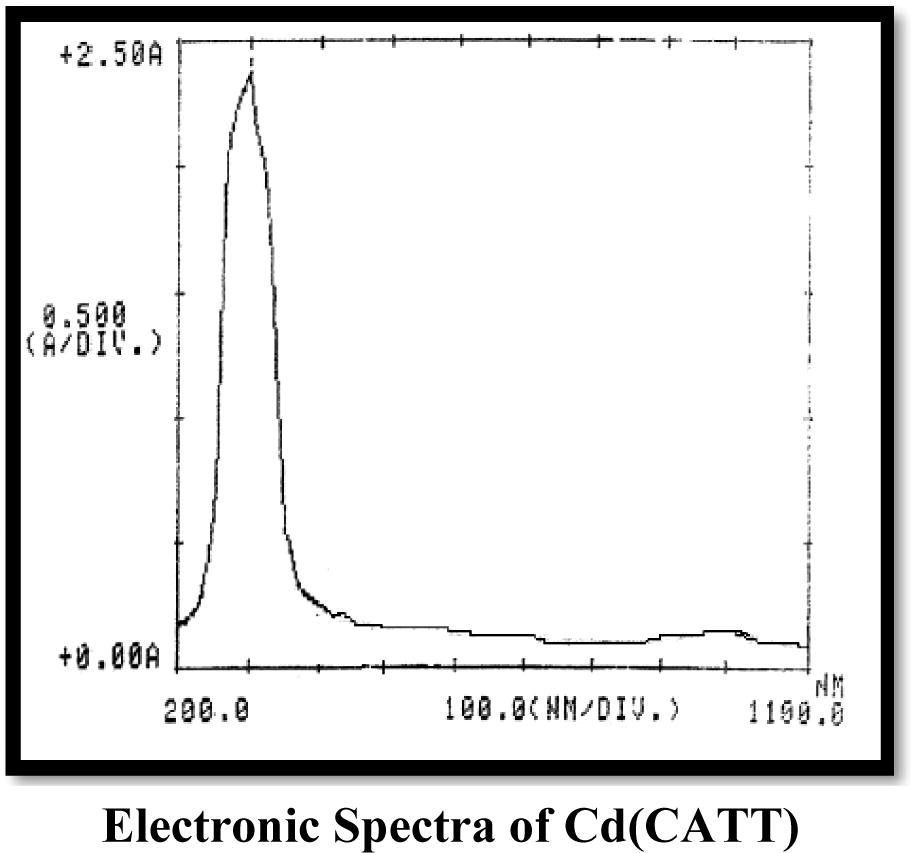
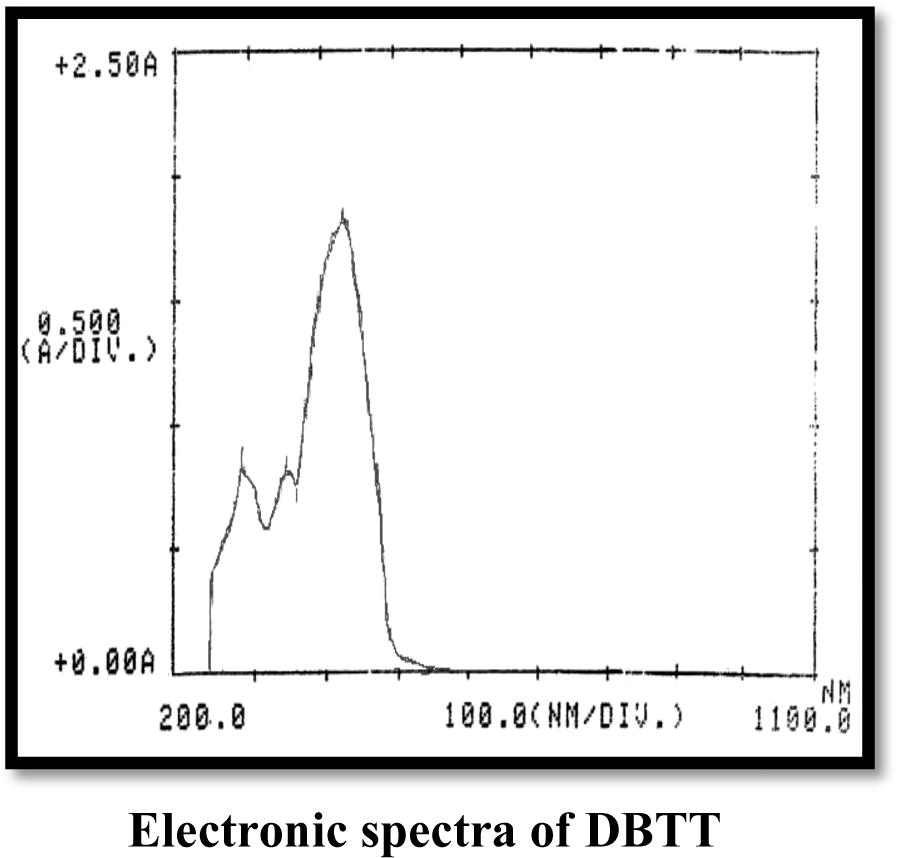
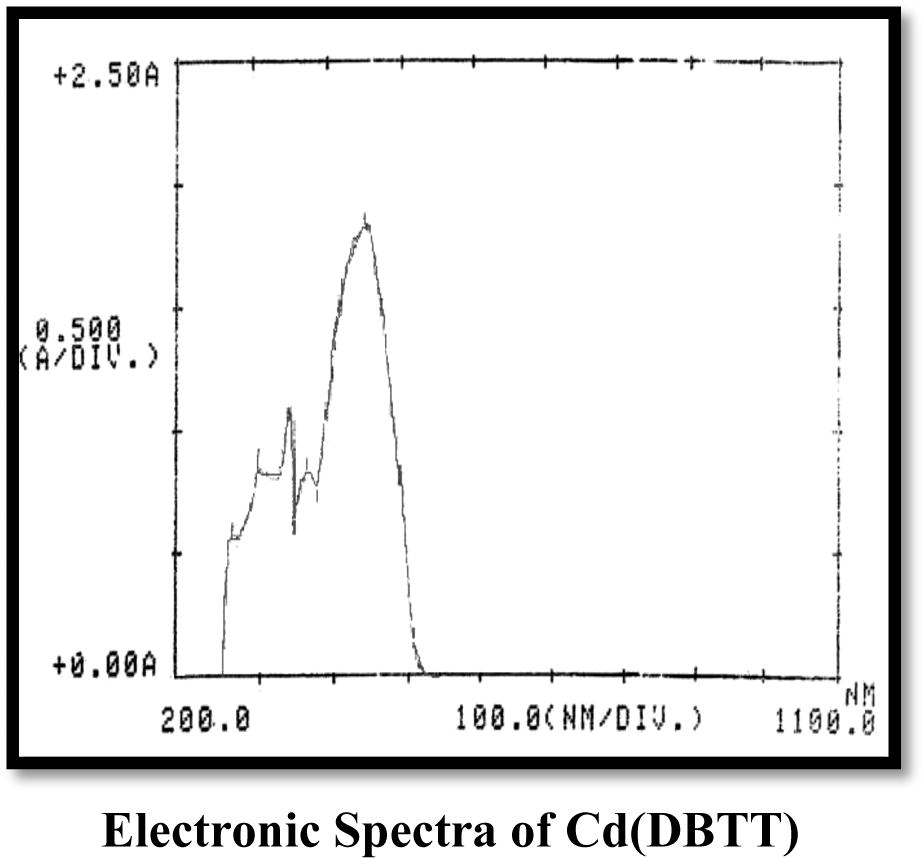
3.3 Thermal analyses
Thermal analyses by the TGA technique has proved to be very useful in determining the crystal water or ethanol content in complexes and their thermal stability and decomposition mode under a controlled heating rate. The weight loss was measured from 35 to 900°C at 20.00°C/min. In the Cd(CATT)2 complex the decomposition started at 75°C and was completed at 795°C. A mass loss occurred within the temperature range 75–220°C, corresponding to three uncoordinated ethanols. Another two steps observed in the temperature range of 220–650 and 650–795°C refer to the decomposition of 2C5H4N3O2S and CdS6, respectively.
The Mn(CATT)2 complex was stable up to 70°C and its decomposition started at this temperature and was completed at 845°C. A mass loss occurred within the temperature range of 70–130°C corresponding to the loss of EtOH molecules, 130–250°C refer to aqua H2O molecules as well as two steps at 250–675 and 675–845°C corresponding to the decomposition of 2C5H4N3O2S of the ligand and MnS6, respectively.
The TGA of Fe2(CATT)4 started from 40°C and was completed at 890°C. A mass loss occurred within the temperature range of 40–90°C corresponding to the loss of the outer sphere of water molecules, 90–740°C and 740–890°C refer to the decomposition of 4C5H4N3O2S, Cl2 and 2FeS6, respectively.
Cd(DBTT) shows four steps of weight loss in the temperature range of 50–230, 230–320, 320–760 and 760–880°C. These mass losses are attributed to ethanol molecules, the methyl group of the ligand, C10H5N4S, the Cl of the ligand and CdS3 respectively.
In Mn(DBTT) the first step of mass loss occurred within the temperature range of 40–90°C, corresponding to hydrate molecules, the second step that exhibits in the temperature range of 90–200°C refers to two aqua molecules, as well as the four steps that refer to OAc, 2CH3, the C10H5N4S of the ligand and MnS3 were observed in the temperature range of 200–270, 270–335, 335–790 and 790–900°C respectively (Table 4).
Comp. | Dissociation stages | Temp range in TG (°C) | Weight loss found (Calcd.) (%) | Decomposition assignment |
---|---|---|---|---|
Cd(CATT)2 (782.41) | Stage I | 75–220 | 17.21 (17.63) | 3C2H5OH |
Stage II | 220–650 | 43.61 (43.45) | 2C5H4N3O2S of ligand | |
Stage III | 650–795 | 38.98 (38.90) | CdS6 | |
Mn(CATT)2 (668.93) | Stage I | 70–130 | 6.98 (6.87) | C2H5OH |
Stage II | 130–250 | 5.65 (5.38) | Coordination water molecules | |
Stage III | 250–675 | 50.88 (50.82) | 2C5H4N3O2S of ligand | |
Stage IV | 675–845 | 36.66 (36.91) | MnS6 | |
Fe2(CATT)4 (1282.69) | Stage I | 40–90 | 2.54 (2.80) | 2H2O |
Stage II | 90–740 | 58.87 (58.54) | 4C5H4N3O2S, Cl2 of ligand | |
Stage III | 740–890 | 38.43 (38.64) | 2FeS6 | |
Cd(DBTT) (670.91) | Stage I | 50–230 | 27.35 (27.42) | 4C2H5OH |
Stage II | 230–320 | 4.57 (4.47) | 2CH3 | |
Stage III | 320–760 | 37.31 (37.03) | C10H5N4S, Cl of ligand | |
Stage IV | 760–880 | 31.20 (31.06) | CdS3 | |
Mn(DBTT) (506.93) | Stage I | 40–90 | 7.05 (7.10) | H2O |
Stage II | 90–200 | 3.50 (3.55) | Coordination water molecules | |
Stage III | 200–270 | 11.68 (11.36) | OAc | |
Stage IV | 270–355 | 5.71 (5.91) | 2CH3 | |
Stage V | 355–790 | 42.29 (42.01) | C10H5N4S of ligand | |
Stage VI | 790–900 | 29.88 (29.77) | MnS3 | |
Fe(DBTT) (575.84) | Stage I | 80–210 | 15.88 (15.97) | 2C2H5OH |
Stage II | 210–260 | 3.32 (3.12) | Coordination water molecules | |
Stage III | 260–365 | 5.29 (5.20) | 2CH3 | |
Stage IV | 365–810 | 49.88 (49.31) | C10H5N4S, Cl2 of ligand | |
Stage V | 810–900 | 26.67 (26.36) | FeS3 |
The Fe(DBTT) complex was stable up to 80°C and its decomposition started at this temperature and was completed at 900°C. A mass loss occurred within the temperature range 80–210°C corresponding to the loss of 2EtOH molecules, 210–260°C refer to the coordinated water molecule as well as at 260–365, 365–810 and 810–900°C corresponding to the decomposition of 2CH3, C10H5N4S and MnS6, respectively.
The determined temperature ranges, corresponding percent mass losses and decomposition assignment are given in (Table 4). Data from the thermal analyses sustain the molecular formulas assigned for these complexes. The structural formulas assigned to the complexes are presented in Fig. 1.
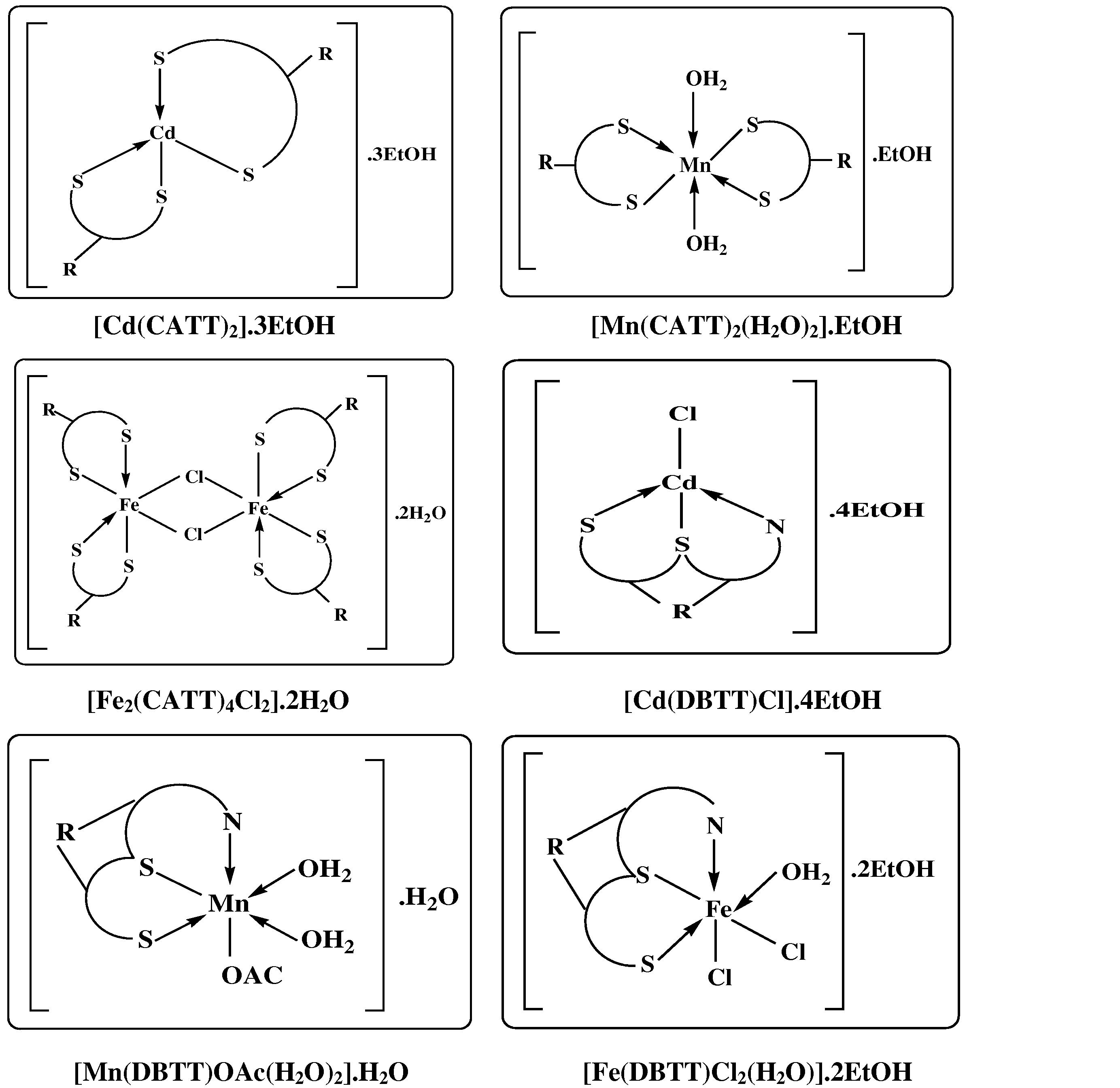
- The suggested structures of prepared new complexes.
3.4 Suggested structure of complexes
See Fig. 1.
3.5 Antibacterial activities
The in vitro antibacterial activity of the synthesized ligands and their corresponding metal complexes on selected bacteria P. aeruginosa and S. aureus was carried out and compared with the standard drug (Ampicillin). On comparing the biological activity of the ligands and their metal complexes with the standard drug, the values indicate that some complexes have higher antibacterial activity than the free ligands. In the Cd(II) complexes at 5 mM concentration the results recorded a higher inhibition zone of Cd(DBTT) and Cd(CATT)2 respectively against P. aeruginosa comparable with the standard drug, the same effect was noticed against S. aureus but with a low inhibition zone comparable with the standard drug, while in 10 mM concentration the results exhibit high biological activity of Cd(CATT)2 against P. aeruginosa, while we noticed that in contrast Cd(DBTT) recorded high biological activity against S. aureus comparable with the standard drug. Figs. 2 and 3 shows that the Mn(CATT)2 complex has better antibacterial activity in (5 mM) concentration against gram negative strain bacteria (P. aeruginosa) and negligible effect against gram positive strain bacteria (S. aureus), while the Mn(DBTT) complex did not record any significant effect toward the two kinds of bacteria in this concentration. In 10 mM concentration the results exhibit a higher inhibition zone of Mn(CATT)2 against P. aeruginosa and a moderate effect against S. aureus, while the Mn(DBTT) complex exhibits the same effect which was noticed in (5 mM) concentration. For Fe(III) complexes only the Fe(DBTT) complex recorded significant activity in high concentration, that is, 10 mM against two selected bacteria and the Fe(DBTT) complex in low concentration against the gram positive strain bacteria (S. aureus) only, whereas the Fe(DBTT) complex did not exhibit any significant effect in this concentration against another type of tested microorganism, that is, P. aeruginosa, and this effect was also observed in Fe2(CATT)4 in both concentrations and both kinds of tested bacteria comparable with the standard drug.
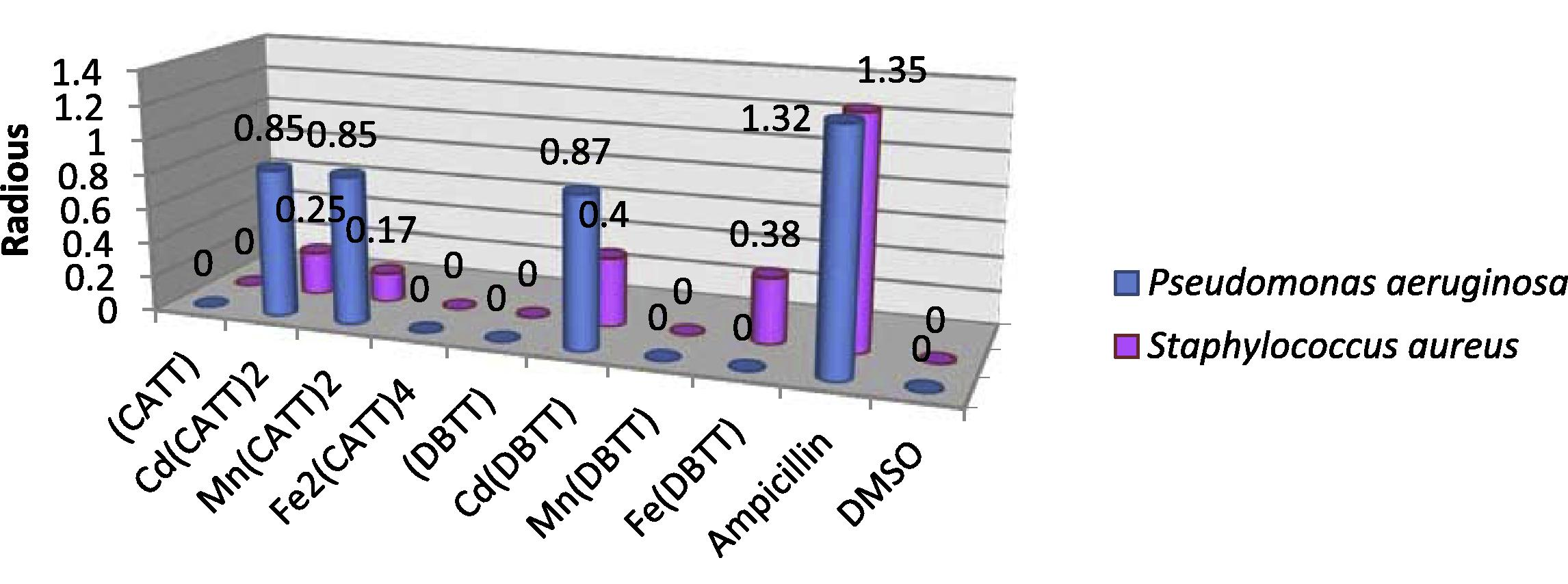
- The effect of ligands and their metal complexes toward Pseudomonas aeruginosa and Staphylococcus aureus at concentration 5 mM.
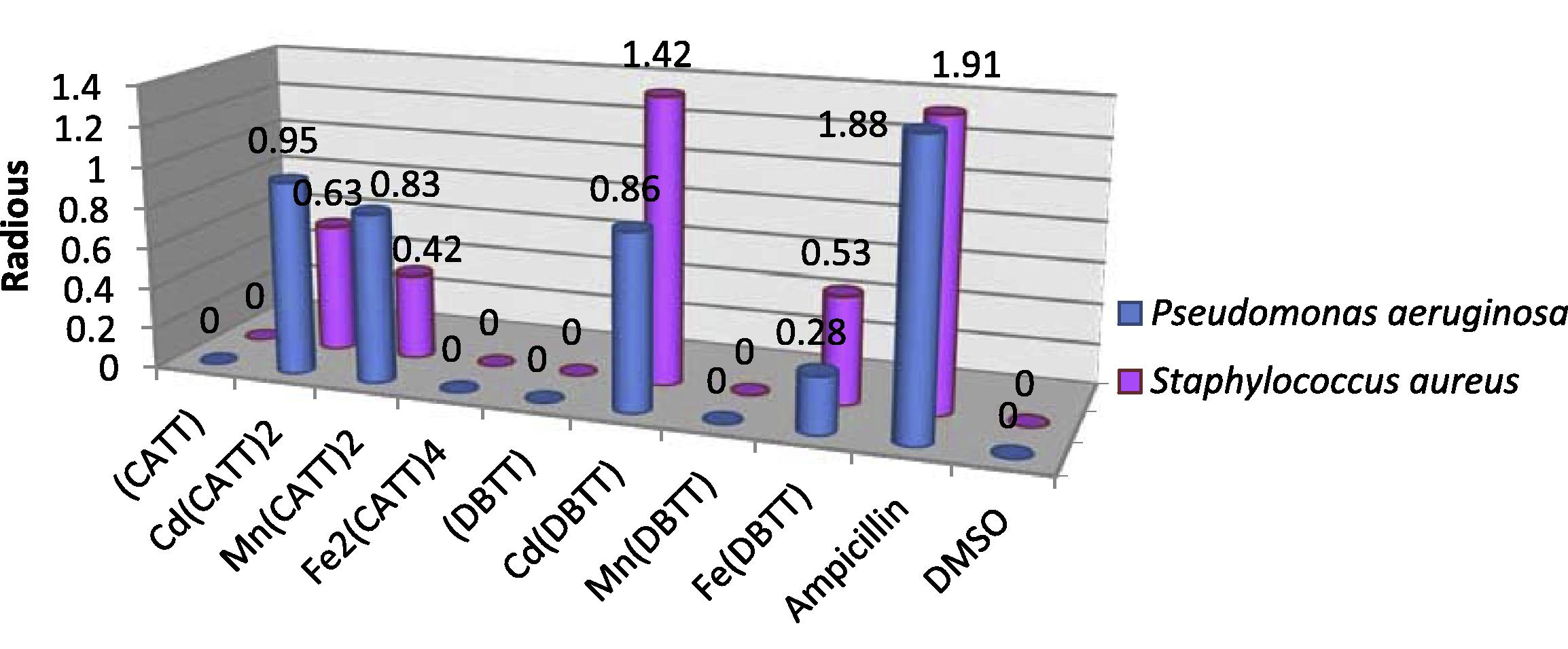
- The effect of ligands and their metal complexes toward Pseudomonas aeruginosa and Staphylococcus aureus at concentration 10 mM.
The higher inhibition zone of the metal complexes than those of the ligands can be explained on the basis of Overtone's concept and the Chelation theory. On chelation, the polarity of the metal ion will be reduced to a greater extent due to the overlap of the ligand orbital and partial sharing of the positive charge of the metal ion with the donor groups. Further, it increases the delocalization of π-electrons over the whole chelating ring and enhances the penetration of the complexes into lipid membranes and blocking of the metal binding sites in the enzymes of microorganisms. There are other factors which also increase the activity such as solubility, conductivity and bond length between the metal and ligand (Mohamed et al., 2009; Chohan et al., 2004; Singh et al., 2008), the antibacterial data listed in (Table 5). The investigation of the antibacterial data revealed that the Mn(II) and Cd(II) complexes of the CATT ligand exhibited better antibacterial activity against P. aeruginosa compared to the other compounds as well as the standard drug ampicillin, while the results show that the Cd(II) of the DBTT ligand display high activity against P. aeruginosa and S. aureus in comparison to other compounds and ampicillin Figs. 2 and 3.
Comp. | Diameter of inhibition zone (mm); concentration in mM | |||
---|---|---|---|---|
Pseudomonas aeruginosa | Staphylococcus aureus | |||
5 mM | 10 mM | 5 mM | 10 mM | |
(CATT) | − | − | − | − |
Cd(CATT)2 | ++ | ++ | + | ++ |
Mn(CATT)2 | ++ | ++ | − | + |
Fe2(CATT)4 | − | − | − | − |
(DBTT) | − | − | − | − |
Cd(DBTT) | ++ | ++ | + | +++ |
Mn(DBTT) | − | − | − | − |
Fe(DBTT) | − | + | + | + |
DMSO | − | − | − | − |
Ampicillin | ++ | +++ | ++ | +++ |
(−) No inhibition; (+) inhibition in diameter (0.25–0.82) mm; (++) inhibition in diameter (0.83–1.37) mm; (+++) inhibition in diameter (1.38–1.93) mm.
4 Conclusion
In the present research study, efforts were made to synthesize some new ligands of trithiocarbonate from 2-amino-5-mercapto1,3,4-thiadiazole. These synthesized compounds were used to prepare some new complexes, and these complexes are characterized by various physicochemical and spectral analyses. The results exhibit that the synthesized ligand CATT binds with metal ions in a bidentate manner, with S,S donor sites of trithiocarbonate, while the DBTT ligand binds with metal ions in tridentate by S,S donor sites of trithiocarbonate as well as the N atom of azomethine. Thermo gravimetric studies of the complexes also helped to characterize the complexes. The antibacterial data show the metal complexes to be more biologically active compared to those of the parent ligands against both microorganism species.
References
- 1H 13C NMR investigation of E/Z-isomerization around C{double bond, long}N bond in the trans-alkene-Pt(II)imine complexes of some ketimines and aldimines. J. Saudi Chem. Soc.. 2010;14(2):165-174.
- [Google Scholar]
- An efficient, one-pot synthesis of trithiocarbonates from the corresponding thiols using the Mitsunobu reagent. Tetrahedron Lett.. 2008;49:4886-4888.
- [Google Scholar]
- Antifungal cobalt(II), copper(II), nickel(II) and zinc(II) complexes of furanyl-, thiophenyl-, pyrrolyl-, salicylyl- and pyridyl-derived cephalexins. J. Enzyme Inhib. Med. Chem.. 2004;19(1):85-90.
- [Google Scholar]
- Dependence of ring-opening reaction of 5-membered dithiocarbonates oncationic catalyst-control of isomerization and polymerization. Macromolecules. 1998;31(8):2454-2460.
- [Google Scholar]
- Trithiocarbonates: exploration of a new head group for HDAC inhibitors. Bioorg. Med. Chem. Lett.. 2007;17(17):4746-4752.
- [Google Scholar]
- Synthesis, characterization and thermogravimetric studies of some metal complexes with N2O2 Schiff base ligand. World J. Chem.. 2010;5(1):57-61.
- [Google Scholar]
- Synthesis and in vitro leishmanicidal activity of 2-(5-nitro-2-furyl) and 2-(5-nitro-2-thienyl)-5-substituted-1,3,4-thiadiazoles. Bioorg. Med. Chem. Lett.. 2005;15(8):1983-1985.
- [Google Scholar]
- Synthesis and characterization of mixed ligand complexes of some metals with 1-nitroso-2-naphthol and l-leucin. Diyala J. Pure Sci.. 2012;8(2):118-131.
- [Google Scholar]
- Microwave assisted synthesis, spectroscopic, thermal and antimicrobial studies of some transition metal complexes of Schiff base ligands containing thiazole moiety. Jordan J. Chem.. 2012;7(1):9-21.
- [Google Scholar]
- Synthesis, physical characterization of M(III) transition metal complexes derived from thiodihydrazide and 5-tert-butyl-2-hydroxy-3-(3-phenylpent-3-yl) benzaldehyde. Eur. J. Chem.. 2012;9(4):2119-2127.
- [Google Scholar]
- In vitro antifungal activity of 2,5 disubstituted amino-oksometyloso-arylo-thiadiazole derivatives. J. Adv. Med. Sci.. 2007;52(1):26-29.
- [Google Scholar]
- Synthesis and dyeing performance of some novel heterocyclic azo disperse dyes. J. Braz. Chem. Soc.. 2001;12(6):1-6.
- [Google Scholar]
- Advanced Organic Chemistry (sixth ed.). Hoboken, New Jersey: John Wiley & Sons; 2007.
- Transition metal complexes of isonicotinoyl-hydrazone-4-diphenylaminobenzaldehyde: synthesis, characterization and antimicrobial studies. Eur. J. Chem.. 2012;9(1):365-372.
- [Google Scholar]
- Biological activity studies on metal complexes of novel tridentate Schiff base ligand. Spectroscopic and thermal characterization. Eur. J. Med. Chem.. 2009;44(12):4801-4812.
- [Google Scholar]
- Infrared and Raman Spectra of Inorganic and Coordination Compounds (sixth ed.). New Jersey: John Wiley & Sons, Inc.; 2009.
- Magnetic and spectroscopic studies of the synthesized metal complexes of bis(pyridine-2-carbo)hydrazide and their antimicrobial studies. Eur. J. Chem.. 2012;9(4):1835-1842.
- [Google Scholar]
- Synthesis, characterization of some transition metal(II) complexes of acetone p-amino acetophenone salicyloyl hydrazone and their anti microbial activity. Biometals. 2008;21(4):491-501.
- [Google Scholar]
- Synthesis and characterization of a novel benziloxime ligand and its iron(III) and nickel(II) complexes. J. Chin. Chem. Soc.. 2010;57(3A):332-337.
- [Google Scholar]
- Inorganic Electronic Structure and Spectroscopy: Applications and Case Studies. Vol vol. 2. New York, Chester, Singapore, Toronto: John Wiley and Sons Inc.; 2006.
- Determination of organotrithiocarbonates with O-diacetoxyiodobenzoate and N-chlorosuccinimide in aqueous and non-aqueous media. Analyst. 1990;115(4):421-423.
- [Google Scholar]
- Complexes with S-donor ligands. Synthesis of the first family of (trithiocarbonato) gold complexes. Crystal structure of [(PPh3)2N][AuCl2(CS3)] J. Inorg. Chem.. 1997;36(25):5735-5739.
- [Google Scholar]
- Synthesis of the first trithiocarbonatogold complex: [N(PPh3)2]2[Au2(μ2-η2-CS3)2]. First crystal structure of a μ2-η2-bridging trithiocarbonato complex. J. Chem. Soc., Chem. Commun.. 1995;7:745-746.
- [Google Scholar]
- Green's Protective Groups in Organic Synthesis (fourth ed.). Hoboken, New Jersey: John Wiley & Sons; 2007.