Anti-obesity and antihyperlipidemic effects of Phaleria macrocarpa fruit liquid CO2 extract: In vitro, in silico and in vivo approaches
⁎Corresponding authors at: Drug Discovery and Synthetic Chemistry Research Group, Department of Pharmaceutical Chemistry, Faculty of Pharmacy, IIUM, 25200 Kuantan, Pahang, Malaysia (Q. Uddin Ahmed); Cooperative Research, Extension, and Education Services (CREES), Northern Marianas College, P.O. Box 501250, Saipan MP 96950, USA (Md. Zaidul Islam Sarker). quahmed@iium.edu.my (Qamar Uddin Ahmed), mdzaidul.sarker@marianas.edu (Md. Zaidul Islam Sarker)
-
Received: ,
Accepted: ,
This article was originally published by Elsevier and was migrated to Scientific Scholar after the change of Publisher.
Peer review under responsibility of King Saud University.
Abstract
Objective
Phaleria macrocarpa fruit has been reported to be effectively used in Malaysia and neighboring countries to prevent obesity. Despite its anti-obesity potential, no research has ever predicted the compound-lipase, compound-HMG-CoA reductase interactions and in vivo investigations to further confirm its anti-obesity properties. Thus, the purpose of this study was to assess the anti-obesity and antihyperlipidemic effects of P. macrocarpa by in vitro, computational (in silico), and in vivo assays.
Method
Initially, fruit was extracted through liquid CO2 and heating under reflux extraction methods to obtain liquid CO2 extracts (LCE-1 and LCE-2) and heating under reflux extract (HRE), respectively. Subsequently, all three extracts were assessed for antioxidant potentials through in vitro bioassays and FTIR analysis to identify different types of functional groups present in the existing bioactive compounds. Successively, the most active fraction (LCE-2) was subjected to GCMS analysis for the identification of lipid lowering and lipase inhibitors through molecular docking approach. Finally, anti-obesity and lipid lowering effects were further confirmed through an in vivo assay using mice.
Result
LCE-2 exhibited higher DPPH (IC50 = 0.172 mg/mL) and FRAP (78.98 AAEmg/g) antioxidant activities and showed more potent peaks in FTIR chromatograms than LCE-1 and HRE. Among the identified tentative bioactive compounds viz. methyl palmitate, palmitic acid, ethyl palmitate, methyl oleate, oleic acid, cis-vaccenic acid, 3-deoxyestradiol and phenol, 2,2′-methylenebis[6-(1,1-dimethylethyl)-4-methyl- were found as an anti-obesity and lipid lowering compounds whose protein–ligand interaction was confirmed by binding affinity, amino acid residues and bonding interactions. Similarly, anti-obesity and lipid lowering findings were also found in a mice model after 6 weeks treatment at a dose of 250 mg/kg b.w.
Conclusion
Based on the aforementioned in vitro, in silico and in vivo findings, it is concluded that the LCE-2 possesses lipase and HMG-CoA reductase inhibitors that can assist to develop this plant’s extract as an alternative safe lipid lowering herbal medicine in future.
Keywords
P. macrocarpa
CO2 extract
Anti-obesity
In vitro
In silico
In vivo
1 Introduction
Globally, obesity has emerged as a serious public health issue that is characterised by the excessive accumulation of fat in the adipose tissues (Anigboro et al., 2021). According to projections made in the World Obesity Atlas 2022, one billion people throughout the world, including one in every five women and one in every seven males, would be obese by the year 2030 (Lobstein et al., 2022). Predisposition to genes and an imbalance in calorie intake compared to energy expenditure are the two main contributors to obesity. It is widely regarded as the most important factor in the development of a wide variety of chronic conditions, including dyslipidaemia, non-alcoholic fatty liver disease, cardiovascular illnesses, hypertension, diabetes, and many others (Saunders et al., 2018). Elevated levels of triglycerides, total cholesterol, Apo B, and LDL-C are among the lipid abnormalities often identified in obese people (Vekic et al., 2019). The effectiveness of certain previously available anti-obesity medications was hindered by several undesirable side effects, such as gastrointestinal disorders and long-term use detrimental effects on organs, such as lungs, kidney, pancreas, and liver (Muller et al., 2022,). Hence, there is an urgent requirement for a safe alternative treatment that is also effective in combating the disease of obesity. Therefore, numerous scientists are interested in anti-obesity and lipid lowering agents from natural products.
Phaleria macrocarpa (Scheff.) Boerl. is a flowering plant which belongs to the Thymelaeaceae family. It is cultivated in Malaysia, Indonesia, and other Southeast Asian tropical countries. Traditionally, leaves of P. macrocarpa are used as herbal remedies for allergy, breast cancer, lung and colon cancers, diabetes, heart disease and infections related to pathogenic fungal and bacterial strains. People drink it as tea with warm boiled water (Djati, 2021). Furthermore, the bark of this tree is also used to prevent colorectal cancer, bacterial and fungal infections, and cardiac diseases (Hanifah et al., 2019). Another typical component of this plant is seed, which is traditionally used to cure microbial infections and cancers and provide antioxidant benefits (Ahmad et al., 2023). P. macrocarpa fruit has been traditionally used to treat obesity, diabetes, cancer, heart disease, allergy etc. (Mia et al., 2022a). Various in vitro and in vivo research studies have earlier confirmed the anti-diabetes, anti-cancer, antihyperlipidemic, anti-allergic, anti-inflammatory effects of the fruit extracts of P. macrocarpa prepared through conventional extraction techniques generally using toxic organic solvents (Andriani et al., 2015; Christina et al., 2022). Moreover, it has been shown that P. macrocarpa fruit contains different types of phytoconstituents namely flavonoids, fatty acid, fatty acid esters, phenolic acid, lignans, xanthonoids, phytosterols, benzophenone, and other phytoconstituents that might synergistically be accountable for hypocholesterolemia and antidiabetic properties (Magdalita et al., 2018). Even though this fruit is widely used by the native population to treat a variety of illnesses, only traditionally prepared extracts made with toxic organic solvents have been thoroughly studied to date to support its traditional use in the treatment of antihyperlipidemic condition without determining its toxicity or suitability for human consumption (Mia et al., 2022a). As a matter of fact, extraction of plant materials using conventional extraction methods have different limitations viz., require huge amounts of organic solvents, prolong extraction time, degradation of bioactive compounds at high temperature and leftover toxic organic solvents in the final extract have been reported to cause deterioration of the quality of extract and toxicities associated with it. Such impurities of bioactive agents cause a risk of several diseases in humans and ultimately limit their therapeutic safe usages (Easmin et al., 2017; Mia et al., 2022b). Hence, the activity and specificity of the obtained compounds are questionable. To overcome the drawbacks and disadvantages associated with the extracts prepared by the conventional extraction techniques, liquid CO2 extract using non-conventional method was used to overcome the problems associated with the extracts prepared through conventional extraction techniques.
Therefore, the present study was aimed to compare the in vitro antioxidant and functional group analysis of P. macrocarpa fruit conventional and non-conventional extracts. Consequently, GCMS identified bioactive compounds of the highest bioactive fraction were further analysed through in silico molecular docking approaches to study the potential therapeutic properties of the identified compounds. Based on the in vitro and in silico findings, we further analysed the anti-obesity and antihyperlipidemic activities using in vivo mice model.
2 Method
2.1 Plant material collection and identification
The P. macrocarpa fresh ripe red fruits were collected from the nursery at Guar Perahu, Bukit Mertajam, Pulau Pinang. A voucher specimen (IIUM/308/15/2/1/NMPC19-1/13) was deposited in the herbarium of the Kulliyyah of Pharmacy, International Islamic University, Kuantan, Pahang, Malaysia. The samples were recognized by the botanist, Dr. Norazian Binti Mohd. Hassan, KOP, IIUM, Malaysia.
2.2 Liquid CO2 extracts (LCE-1, LCE-2) preparation
About 200 gm of the powdered P. macrocarpa fruit was placed in an extraction vessel and steeped in ethanol at two different ratios: 1:1 (LCE-1) and 1:2 (LCE-2). Following that, the liquid CO2 entered the tank to start the extraction. According to Mia et al. (2022c), fruit powder was extracted using the following conditions: 7.0 MPa pressure, 28.7 °C temperature, 200 cycles, and a run period of 600 min. The extract was separated from the liquid CO2 by vaporization in the reboiler. After collecting the extract from the cyclone separator, the ethanol was evaporated using a rotary evaporator and kept at −80 °C for further analysis (Mia et al., 2022c).
2.3 Heat reflux extract (HRE)
Heat reflux extraction process was followed by the Pudziuvelyte et al. (2018) with some modifications. About 30 gm of P. macrocarpa pulverized fruit flesh powder was dissolved in ethanol (95%) and heated at 95 °C for 120 min followed by filtration using Whatman number 1 filter paper. The remaining residue was subjected to the same condition until no colour remained and was then evaporated using a rotary evaporator (Mia et al., 2022c).
2.4 Antioxidant measurement
2.4.1 Ferric reducing antioxidant power assay
The ferric reducing antioxidant power (FRAP) assay was performed using the method described by Aryal et al. (2019). About 20 μL of P. macrocarpa fruit extracts (1 mg/mL) and different concentrations of ascorbic acid (6.25 – 400 μg/mL) were taken in a 96 microwell plate and added 40 μL of previously prepared FRAP solution. Subsequently, 140 μL of distilled water was added to each test well and mixed gently for a few seconds to produce a blue colour. Blank reagent was prepared by adding 20 μL of extract solvent, 40 μL of FRAP solution, and 140 μL of distilled water for calculation of the test samples. This microwell plate was kept in a dark place for 20 min at a laboratory room temperature. Absorption was measured at 593 nm and calculated the experimental sample as ascorbic acid equivalent per gram (AAE mg/g).
2.4.2 DPPH free radical scavenging activity
With a few minor adjustments, the approach described by Alam et al. (2017) was used to quantify the radical scavenging activity. The solvent, methanol, was used to prepare samples at various concentrations ranging from 2000 to 62.5 µg/mL. In a 96 microwell plate, 80 µL of freshly prepared DPPH (0.1 mM) was first added, and 20 µL of various extract concentrations were subsequently added. The ascorbic acid was used as a positive control that was prepared using methanol. The absorbance was then determined at 517 nm after being left in the dark for 10 min at room temperature.
2.5 Fourier transform infrared spectroscopy (FTIR)
The FTIR- attenuated total reflectance (ATR) measurement of the experimental extracts was performed according to the method described by Jiang et al. (2019) with slight modifications. The samples' FTIR spectra were measured in a spectrum two model instrument at room temperature (25 °C) utilizing ATR and a diamond internal reflection element (PerkinElmer FTIR spectrometer-C98271, USA). The spectral range covered by the instrument was 4000–400 cm−1, with a resolution of 4 cm−1. Before running the samples, the method of ATR scan was utilized directly in this study for each sample. In this experiment, the freeze-dried extracts were kept at room temperature and evaluated on the diamond ATR crystal, without any prior processing.
2.6 Detection of compounds by gas chromatography–mass spectrometry (GC–MS)
GC–MS analysis of the LCE-2 fraction was performed to detect the compounds according to the Nokhala et al. (2020) technique using Agilent 6890 N2 gas chromatography coupled with mass selective detector MS-5973 (model no. 19091S-433). Later, the NIST/EPA/NIH (NIST MS Search version 2.0) mass spectroscopy database was used to identify the compounds.
2.7 In silico molecular docking analysis
The crystallographic structure of the HMG-CoA reductase (1HWK) and pancreatic lipase (1LPB) was downloaded from protein data bank (https://www.rcsb.org/structure/1HWK; https://www.rcsb.org/structure/1LPB). The three-dimensional structure of controls (atorvastatin and orlistat) and identified compounds were downloaded as.sdf format from PubChem database (https://pubchem.ncbi.nlm.nih.gov/) Pubchem-SID (2022). Subsequently, Molecular docking of all identified compounds was performed using sophisticated tools viz. Chimera (version1.15) and PyRx 0.8 (Karale et al., 2022). Later, three-dimensional visualisation and presence of amino acid residues with different bonding interactions was shown using PyMOL (PyMOL2 version 2.4.1) and Biovia Discovery Studio Visualizer (San Diego, CA, USA), respectively (Nipun et al., 2021).
2.8 Animal study
2.8.1 Preparation and acclimatization of mice
Pathogen-free ICR male experimental mice weighing 28–34 g and aged 6–7 weeks were purchased from “A-sapphire enterprise Ltd., Malaysia” for this study. All animals were housed in an SPF-grade laboratory with constant humidity (40–70%) and room temperature (22 ± 2 °C) on a light–dark cycle of 12 h. Mice were housed in sterile polypropylene cages with a basic feed and filtered water. The animals were acclimated for seven days prior to receiving treatment. IACUC approved the animal protocol, as indicated by the ethical clearance reference number IIUM/504/14/2/IACUC. The research was conducted at the animal laboratory, Kulliyyah of Pharmacy, IIUM, Malaysia.
2.8.2 Induction of obesity and treatment
After the acclimatization stage, mice were divided randomly into 6 groups (G1-G6), each group contained seven mice (n = 7). Normal control untreated group was referred to as G1 that received only water and normal diet. Normal control treated group (G2) received water, normal diet and 250 mg/kg b.w. LCE-2. High fat diet (HFD) control untreated group was designated as G3. HFD treated group (G4) received HFD and 250 mg/kg b.w. LCE-2. Sucrose control group (G5) received 20% sucrose ad libitum and normal diet. Sucrose treated group (G6) received 20% sucrose ad libitum, normal diet, and 250 mg/kg b.w. LCE-2. Body weight was recorded weekly during the study period of 30 weeks. After 24 weeks, 250 mg/kg b.w. of LCE-2 were fed to all mice in groups G2, G4 and G6 for 6 weeks. The dose at 250 mg/kg b.w. was considered a safe dose based on our acute and sub-acute toxicity findings of LCE-2 (Mia et al., 2022b).
2.8.3 Analysis of biochemical parameters in blood serum
The biochemistry assay was performed using an auto analyser (Beckman Coulter AU480 Automatic Analyser, Germany). After passing the control, the sample was run for lipid profiles- total cholesterol (TC), high density lipoprotein cholesterol (HDL-C), low density lipoprotein cholesterol (LDL-C) and triglyceride (TG) contents.
2.9 Statical analysis
GraphPad Prism (version 7.00) was used to conduct one-way and two-way ANOVAs, as well as multiple comparison tests, to assess whether there were statistically significant differences between the groups of data.
3 Result
3.1 Yield of extraction
In this research, the liquid CO2 extraction technique was carried out at subcritical pressure (70 bar), temperature (28.7 °C), run time (600 min), 200 cycles. In liquid CO2 extraction, the optimized yield of LCE-1 and LCE-2 was 3.52 ± 0.23% and 4.26 ± 0.21%, respectively. On the other hand, the yield of heat reflux extract (HRE) was 18.21 ± 1.05%.
3.2 Ferric reducing antioxidant power (FRAP) assays
This technique mainly focuses on the overall antioxidant power of the sample, considering the possibility of its oxidation–reduction effect. The findings of the FRAP assay were represented as AAEmg/g of the sample. The LCE-2 revealed the highest FRAP value of 78.98 ± 0.685 AAEmg/g whereas LCE-1 and HRE extracts exhibited values of 41.76 ± 0.442 AAEmg/g and 31.7 ± 0.653 AAEmg/g, respectively. The calibration curve of ascorbic acid standard for FRAP assays was plotted with R2 as 0.9747.
3.3 2,2-Diphenyl-1-picrylhydrazyl (DPPH) free radical scavenging assay
Based on the findings, the LCE-2 was found to exhibit the highest radical scavenging activity among all the tested concentrations, with a value of IC50 = 0.172 mg/mL when compared to the standard, ascorbic acid, which showed a radical scavenging activity with a value of IC50 = 0.015 mg/mL. This was followed by the HRE and LCE-1 extracts of P. macrocarpa’s fruit i.e., IC50 = 0.21 mg/mL and IC50 = 0.23 mg/mL, respectively.
3.4 Fourier transform infrared spectroscopy (FTIR) analysis
The FTIR is a quick analytical method for analysing bioactive substances in natural products. Fig. 1 and Table 1 depict the spectra of LCE-1, LCE-2 and HRE of P. macrocarpa fruit extracts that represent the functional groups. In comparison, it can be observed that the peak at range 3200–3400 cm−1 indicating the presence of hydroxyl group stretching is more prominent in the LCE-2 than LCE-1 as the polarity of the solvent increases.
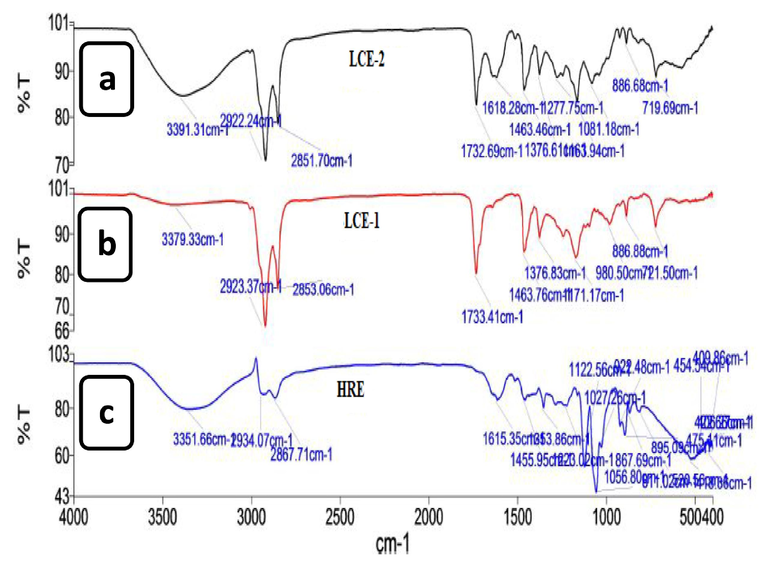
- FTIR spectra of (a) liquid CO2 extract-2 (LCE-2), (b) liquid CO2 extract-1 (LCE-1) and (c) heating under reflux extract (HRE).
Wavenumber (cm−1) | Functional Group | Vibration Mode |
---|---|---|
3200–3400 | O–H (alcohol, carboxylic acid, phenols) | symmetric stretch |
2920–2935, 2850–2870 |
C–H, CHO (alkenes, alkanes, aromatic or aldehydes) |
asymmetric and symmetric stretching of methylene group |
1720–1740 | C = O (ester, ketone, carboxylic acid, or aldehyde) | symmetric stretching |
1610–1620 | N–H (NH2), C = C (amides, amines, alkene) | stretching, bending |
1400–1550 | C = C, N = O (NO2), C-O, C–C (aromatic, alkanes, alkenes, nitro) |
stretching, bending |
1300–1380 | S = O (sulfones, sulfates, sulfonamides, sulfonyl chlorides) | stretching |
1043–1273 | N = O (Nitro) | stretching |
900–1200 | C = O (alcohol, ethers, esters, carboxylic acids, anhydrides) | stretching (carbohydrate region) |
430–800 | -C–H (alkenes, aromatics) | bending and out of plane vibration |
3.5 Identification of bioactive compounds by GC–MS
The LCE-2 fraction was subjected to GC–MS analysis to identify the tentative bioactive compounds present in it. The following nine compounds were verified by comparing the samples' spectrum to the NIST/EPA/NIH mass spectral library's search tool. The results of the peak areas were expressed as peak area counts. The result and chromatogram are shown in Table 2 and Fig. 2, respectively.
Identified compounds | Formula | Mass | Actual mass | Retention time | Area |
---|---|---|---|---|---|
Methyl palmitate | C17H34O2 | 270.5 | 270.2558 | 16.51 | 135,981 |
Palmitic acid | C16H32O2 | 256.42 | 256.2402 | 16.88 | 1,507,707 |
Ethyl palmitate | C18H36O2 | 284.5 | 284.2715 | 17.15 | 158,582 |
Methyl oleate | C19H36O2 | 296 | 296.2715 | 18.16 | 227,170 |
Oleic acid | C18H34O2 | 282.5 | 282.2558 | 18.53 | 1,905,058 |
cis-vaccenic acid | C18H34O2 | 282.5 | 282.2558 | 18.74 | 510,392 |
3-Deoxyestradiol | C18H24O | 256.40 | 256.1827 | 19.89 | 219,885 |
Phenol, 2,2′-methylenebis[6-(1,1-dimethylethyl)-4-methyl- | C23H32O2 | 340.5 | 340.2402 | 20.92 | 199,850 |
Cis-oleic acid | C18H34O2 | 282.5 | 282.2558 | 21.82 | 41,111 |
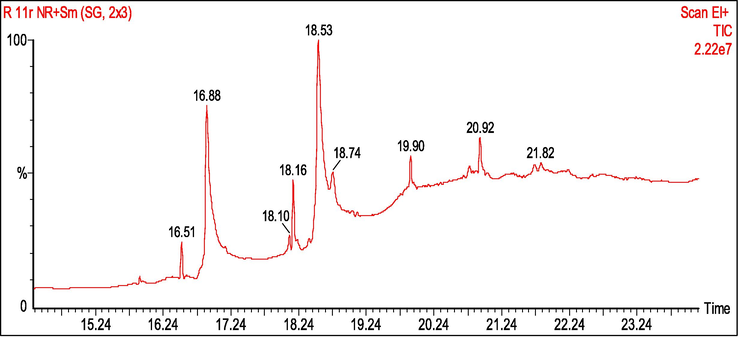
- GC–MS chromatograms of the liquid CO2 extract-2 fraction.
3.6 In silico study of the identified compounds by GC–MS
Molecular docking approach was taken into account to evaluate the probable binding interaction with the targeted receptor to understand the mechanism of action that might explain the inhibitory activities of the eight putative compounds namely methyl palmitate, palmitic acid, ethyl palmitate, methyl oleate, methyl oleate, oleic acid, cis-vaccenic acid, 3-deoxyestradiol and phenol, 2,2′-methylenebis[6-(1,1-dimethylethyl)-4-methyl- identified using GC–MS analyses. According to the docking results, the values of binding affinity (kcal/mol) of the 8 compounds, as well as the positive controls (orlistat and atorvastatin) towards the pancreatic lipase (PDB ID: 1LPB) and HMG-CoA reductase (PDB ID: 1HWK) are shown in Table 3. In compounds-1LPB interactions, compounds namely 3-deoxyestradiol, phenol, 2,2′-methylenebis [6-(1,1-dimethylethyl)-4-methyl-, methyl oleate, palmitic acid, hexadecanoic acid methyl ester were found to exhibit higher binding affinity than the positive control (orlistat) whereas rest of the compounds displayed lower binding affinity than the positive control. The hydrogen and hydrophobic bond interactions with their amino acid residues of all the compounds are shown in Fig. 3. On the other hand, in compound-1HWK interaction, only 3-deoxyestradiol (-7.8 Kcal/mol) and phenol, 2,2′-methylenebis [6-(1,1-dimethylethyl)-4-methyl- (-7.2 Kcal/mol) were found to reveal good binding affinity compared to the positive control viz. atorvastatin (-7.8 Kcal/mol) that might have happened due to the presence and absence of hydrogen and hydrophobic bond interaction with the amino acid residues (Fig. 4).
Identified compounds |
1LPB Binding affinity (Kcal/mol) |
1HWK Binding affinity (Kcal/mol) |
---|---|---|
Methyl palmitate |
−5.9 | −5.6 |
Palmitic acid |
−6.0 | −5.2 |
Ethyl palmitate |
−4.4 | −4.7 |
Methyl oleate | −6.3 | −5.4 |
Oleic acid |
−4.8 | −4.7 |
Cis-vaccenic acid | −5.3 | −5.8 |
3-Deoxyestradiol | −9.4 | −7.8 |
Phenol, 2,2′-methylenebis[6-(1,1-dimethylethyl)-4-methyl- | −8.9 | −7.2 |
Orlistat (Control) | −5.5 | −5.3 |
Atorvastatin (Control) | −7.8 |
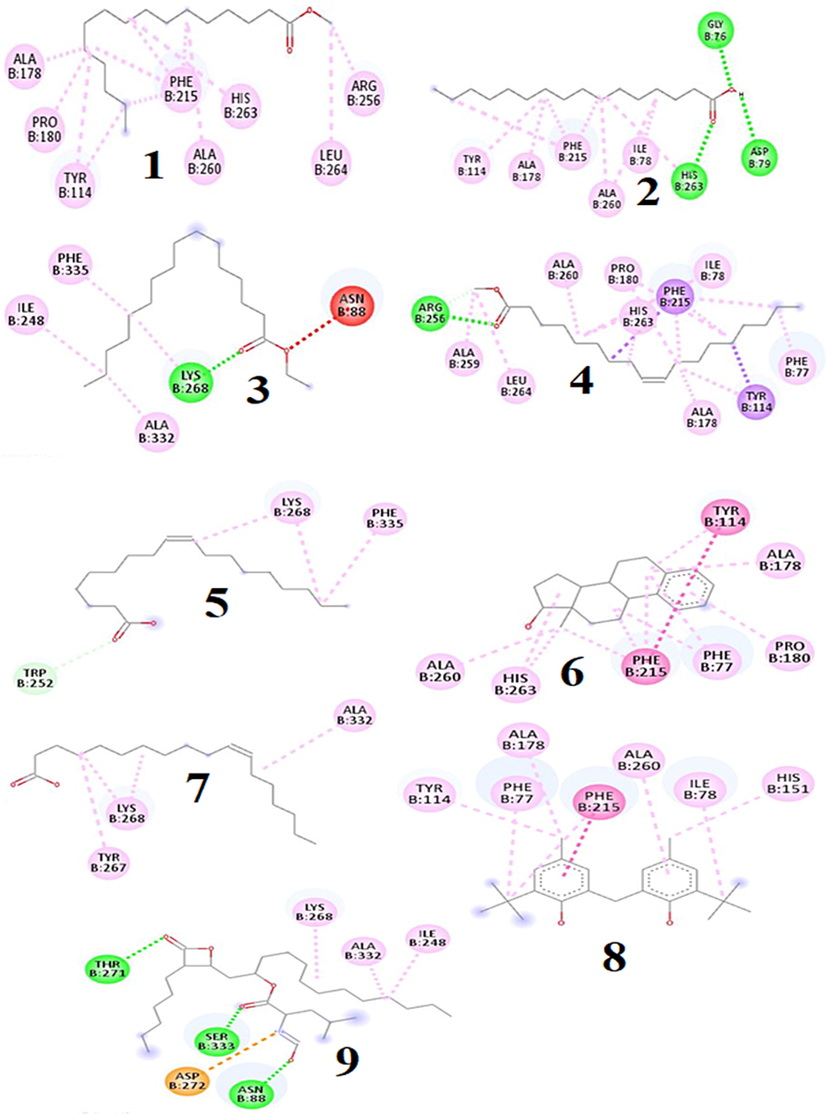
- 2D diagram of compounds (1–8) and control orlistat (9) binding interaction towards the 1LPB enzyme. 1. Methyl palmitate, 2. Palmitic acid, 3. Ethyl palmitate, 4. Methyl oleate, 5. Oleic acid, 6. cis-vaccenic acid, 7. 3-Deoxyestradiol, 8. Phenol, 2,2′-methylenebis[6-(1,1-dimethylethyl)-4-methyl-, 9. Orlistat (Control).
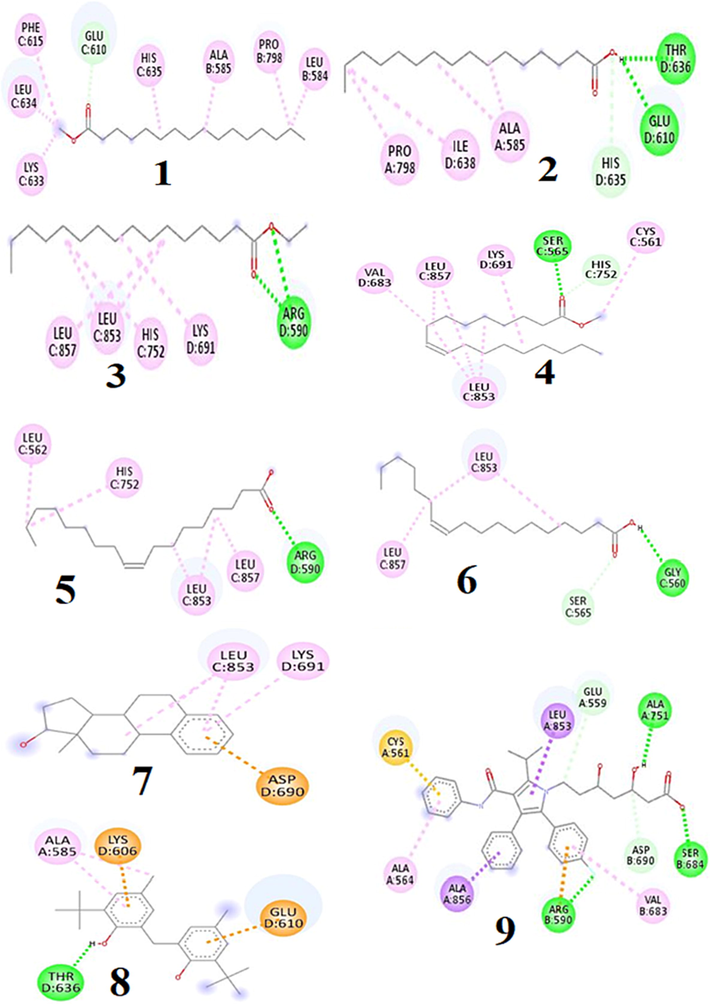
- 2D diagram of compounds (1–8) and control atorvastatin (9) binding interaction towards the 1HBK enzyme. 1. Methyl palmitate, 2. Palmitic acid, 3. Ethyl palmitate, 4. Methyl oleate, 5. Oleic acid, 6. cis-vaccenic acid, 7. 3-Deoxyestradiol, 8. Phenol, 2,2′-methylenebis[6-(1,1-dimethylethyl)-4-methyl-, 9. Atorvastatin (Control).
3.7 Body weight
The body weight was increased until 24 weeks. All the LCE-2 treated groups showed a decreased body weight after week 24 (Fig. 5). Between weeks 24 to 30, the body weight increased 10.19%, 9.67% and 7.72% in groups G1, G3 and G5, respectively. Whereas weight loss was observed by 3.95%, 15.89% and 11.06% in LCE-2 treated groups G2, G4 and G6, respectively.
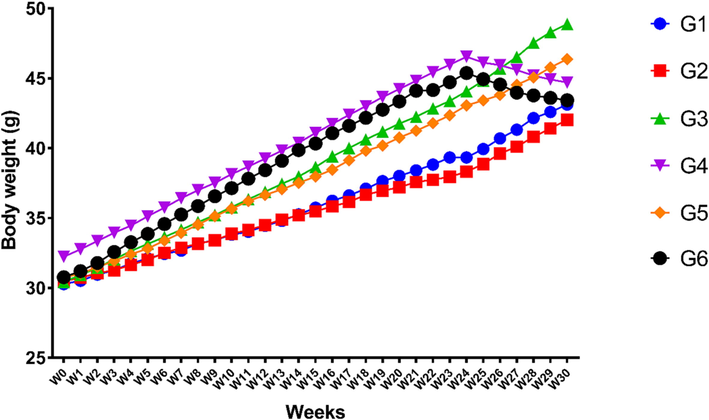
- Status of body weight in different experimental groups.
3.8 Effect of LCE-2 on serum lipids
The total cholesterol was significantly higher i.e., 63.16% in the high fat treated group (G3) compared to the normal control G1. LCE-2 treated groups showed significantly lower cholesterol level G3 vs G4 (p < 0.001). Total cholesterol level was slightly higher in the G5 group, but this was significantly lower in a group that consumed 20% sucrose with LCE-2 (G5 vs G6, p < 0.01) (Fig. 6A). In all the LCE-2 treated groups, LDL level was found to be significantly lowered i.e., 24.82%, 56.42%, and 40.69% compared to the respective comparing groups G1 vs G2, G3 vs G4 (p < 0.001), and G5 vs G6 (p < 0.05), respectively (Fig. 6B). LCE-2 treatment groups showed elevated HDL levels. The difference was significantly higher i.e., 52.80% and 21.87% between the LCE-2 treated high fat diet fed group G3 vs G4 (p < 0.001) and 20% sucrose induced group (G5 vs G6), respectively (Fig. 6C). LCE-2 treated (250 mg/kg bw) G4 and G6 groups were found to have significantly decreased TG level i.e., 33.53% (p < 0.001) and 26.22% (p < 0.001) compared to the diet control groups G3 and G5, respectively (Fig. 6D).
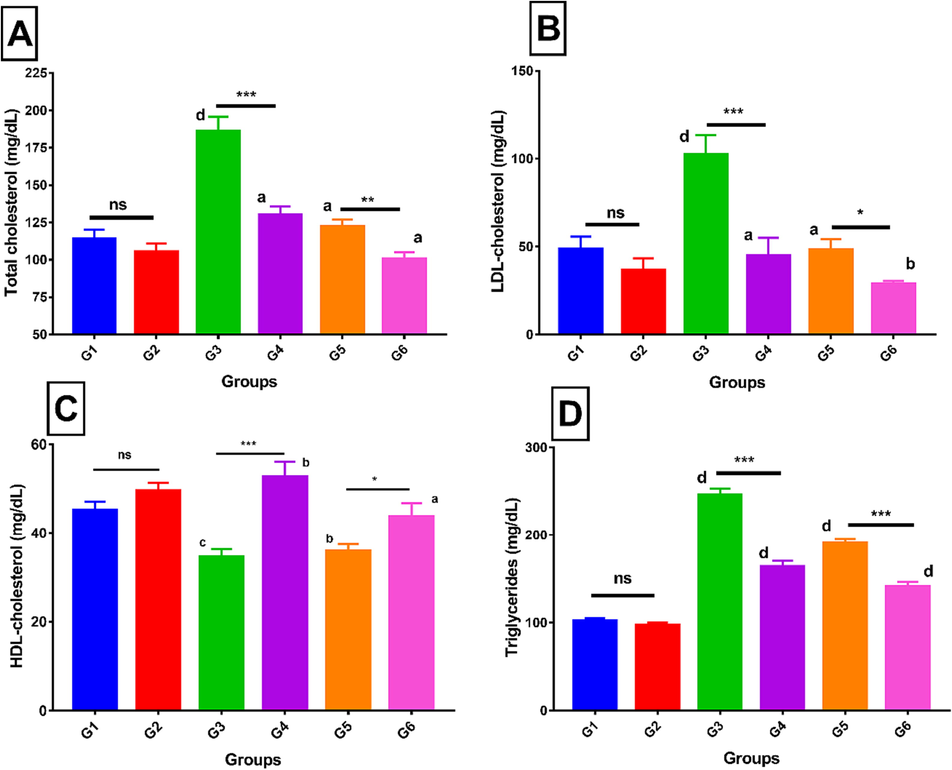
- A. Total cholesterol of different experimental groups; B. Comparison of serum LDL-cholesterol level between different experimental groups; C. Serum HDL-cholesterol comparison with different experimental groups; D. Serum triglycerides comparison with different experimental Values are means ± SEM (n = 7). ns is non-significant, *p < 0.05, **p < 0.01 and ***p < 0.001 significantly different from the same diet control group. Small letters (a, b, c, d) represent the comparative analysis with control untreated (G1), “a” is the non-significant, b (p < 0.05), c (p < 0.01) and d (p < 0.001) were the significant different from control untreated (G1).
4 Discussion
The search for antihyperlipidemic and anti-obesity drugs is of great interest among the researchers, as the disease has been reported to affect a lot of people throughout the world (Pulipati & Pannain, 2022). Based on the traditional claim of P. macrocarpa fruit as an anti-obesity agent, it was scientifically analysed through antioxidant, molecular docking with two enzymes (1HWK and 1LPB) and finally the traditional claim was further confirmed through body weight measurement and lipid profile analysis of mice. Antioxidants are considered good for the body because they get rid of free radicals, which can help reduce cholesterol levels and keep a person free of having different disorders (Akbari et al., 2022). Natural antioxidants also act by inhibiting the propagation step of lipid peroxidation (Farhoosh, 2022). In this research, LCE-2 showed a strong FRAP and DPPH antioxidant activities compared to the LCE-1 and HRE fractions. In this study, LCE-2 had a higher DPPH activity, which could be easily explained by the non-traditional extraction method and the use of food-grade ethanol as an extraction solvent. Babova et al. (2016) found that food-grade ethanol increases the solubility of CO2 and the amount of flavonoid/polyphenol-rich extract.
The FTIR is a quick analytical method to identify compounds by confirming the presence of key functional groups present in these substances. It was revealed that the different functional groups were present in LCE-2, LCE-1 and HRE. In comparison, it can be observed that the peak at range 3200–3400 cm−1 indicating the presence of hydroxyl group stretching is more prominent in the LCE-2 than LCE-1 as the polarity of the solvent increases. Besides that, LCE-2 and LCE-1 showed a more visible C–H stretching peak at range from 2850 to 2935 cm−1 than HRE which occurs due to the presence of alkanes, alkenes, and aldehydes related. Since more hydroxyl group, amide group and other peaks were more prominent in LCE-2 than other fractions (LCE-1 and HRE) that could be responsible for more bioactivities and have the chance to get enriched lipase and HMG-CoA reductase inhibitory bioactive compounds than other fractions (Aqeel et al., 2018). So, based on the highest antioxidant activity and prominent FTIR peak analysis, LCE-2 fraction was selected for an in vivo evaluation to further confirm its antihyperlipidemic and anti-obesity potentials. LCE-2 was also subjected to GC–MS analysis to identify bioactive compounds present in this extract. Compounds namely methyl palmitate and ethyl palmitate have been reported to play an important role in stimulating pancreatic activity. Moreover, these compounds have also been reported to activate the GP/PPARα that are involved in lipid metabolism through transcriptional regulation (Choudhary et al., 2019). Results revealed that palmitic acid was one of the isolated compounds from Ximenia americana that exhibited a strong antioxidant activity (Shettar et al., 2017). Oleic acid has been shown to reduce hypercholesterolemia and oxidative stress in individuals with improved serum lipoprotein profile (Priore et al., 2017). When tested on diabetic patients, vaccenic acid had significant hypotriglyceridemic effects (Wang et al., 2008). Plant antioxidants have a high potential for improving antihyperlipidemic, antiobesity, and protecting the body from increased oxidative stress in diabetics (Sun et al., 2018). Based on the reported bioactivity of tentative compounds found by GCMS, we can say that LCE-2 has a strong antioxidant effect. Furthermore, in silico analysis was performed to understand the interactions of all the identified bioactive compounds with the targeted receptors i.e., HMG-CoA reductase and pancreatic lipase to understand their role as antihyperlipidemic and anti-obesity agent.
According to the research findings, blocking HMG-CoA reductase and pancreatic lipase has been the most effective way to treat both hyperlipidemic and obesity disorders respectively (Gandhi et al., 2019; Reddy et al., 2019). Eight identified GC–MS compounds were evaluated through molecular docking analysis to predict the compound-1LPB and compound-1HWK interactions. The identified compounds firmly fit into the active binding sites of HMG-CoA reductase and lipase enzyme through different hydrophilic and hydrophobic interactions that were comparable to the docking outcome of atorvastatin and orlistat, respectively (Figs. 5 and 6)). Similar findings were also reported by other research studies (Gandhi et al., 2019; Aqeel et al., 2018).
Among dietary foods, higher carbohydrate and fat intake are the two parameters that are linked to obesity (Shatwan & Almoraie, 2022). According to the findings (Fig. 5), the body weight was significantly increased in all groups (G1-G6) before treatment until 24 weeks. Interestingly, continuous supplementation of the LCE-2 extracts for six weeks (week 24–30) considerably decreased the weight gain as compared to the HFD control (G3) and sucrose group (G5). Without altering the amount of food consumed, the LCE-2 therapy prevented weight increase, suggesting its ability to prevent weight gain without altering satiety. Similar findings were also reported using different solvent extracts by other researchers (De Campos et al., 2021). Lipid profile is a reliable predictor of the onset of issues associated with metabolic diseases caused by obesity (Rohm et al., 2022). A profound impact of LCE-2 on lipid profile of the mice was observed. While total cholesterol was significantly decreased in the treated groups (G4 and G6) compared to the respective control groups (G3 and G5). To further insight into the changes in their lipid profile, the serum LDL level was also quantified. In this experiment, an anticholesteremic effect of LCE-2 was also observed. The serum HDL level (also called as good cholesterol, that helps to reduce circulatory LDL level) was next assessed. Interestingly, it was observed that LCE-2 (250 mg/kg b.w.) treatment groups consistently had higher HDL levels. Finally, the circulatory triglycerides (TG) were assessed in these animals to confirm the anti-lipogenic effect of LCE-2. The findings suggested an anti-lipogenic effect of LCE-2 that has been reported by another researcher (Park et al., 2020). LCE-2 (250 mg/kg b.w.) therapy in G4 and G6 groups resulted in serum lipid normalisation, which may have contributed to the favourable impact on pancreatic cells. Previous research studies revealed that P. macrocarpa fruit aqueous extract treated animals had significantly reduced LDL-cholesterol, triglycerides, total cholesterol, and the same extract also up-regulated both the LDL receptor and PCSK9 at protein and mRNA levels (Berlian et al., 2018). In addition, another research study also showed that the fruit extract prepared through conventional extraction technique using toxic ethyl acetate solvent at doses 500 mg/kg b.w. reduced cholesterol level (Andriani et al., 2015). Whereas, in our current study, it was revealed that liquid CO2 extract at a dose 250 mg/kg b w. possessed antidyslipidemic effect which was manifested by reducing cholesterol, triglycerides, LDL-cholesterol as well as increasing HDL-cholesterol in the blood that might have happened due to the selection of extraction techniques, nature of solvent and conditions were set up.
5 Conclusion
P. macrocarpa is found to be one of the most popular medicinal plants traditionally used to treat diabetes and other ailments in Malaysia and other Southeast Asian tropical countries. This research has proved the antihyperlipidemic potential of P. macrocarpa fruit through in vitro, in silico and in vivo assays. It was found out that LCE-2 showed higher antioxidant activities and potent functional groups than HRE and LCE-1 extracts. Although, all compounds identified through GC–MS were found to possess good to high binding affinity and binding interaction compared to the controls for both HMG-CoA reductase and pancreatic lipase. Among the all identified compounds, 3-deoxyestradiol and phenol, 2, 2′-methylenebis[6-(1,1-dimethylethyl)-4-methyl- were found to display the best docking effect score that might be attributable to a potent anti-obesity and antihyperlipidemic effects of LCE-2 extract. Subsequently, LCE-2 extract also exhibited good in vivo antihyperlipidemic and anti-obesity effects. Overall, our findings confirmed that LCE-2 is an abundant source of natural antioxidant, anti-obesity and antihyperlipidemic agents which could be optimized for development of new anti-obesity and antihyperlipidemic drugs. However, some more studies for future research studies particularly efficacy as well as toxicological effects of all individual bioactive compounds as active principles are still required prior to recommending human clinical trials for the suitability of this plant as antihyperlipidemic and anti-obesity agent.
Institutional review board statement
The animal handling research protocol was initially approved by the IIUM ANIMAL CARE AND USE COMMITTEE (I-ACUC), with the ethical clearance reference number IIUM/504/14/2/I-ACUC.
Data availability statement
Data is available in the article.
Acknowledgments
This work was funded by Researchers Supporting Project number (RSPD2023R728), King Saud University, Riyadh, Saudi Arabia. The authors are also thankful to Joint Research Project (UMP-IIUM-UiTM Sustainable Research Collaboration Grant 2020, Malaysia; ID: SRCG20-002-0002) and Jeffrey Cheah School of Medicine and Health Sciences, Monash University Malaysia.
Declaration of Competing Interest
The authors declare that they have no known competing financial interests or personal relationships that could have appeared to influence the work reported in this paper.
References
- Phaleria macrocarpa (Scheff.) Boerl.: An updated review of pharmacological effects, toxicity studies, and separation techniques. Saudi Pharm. J.. 2023;31(6):874-888.
- [Google Scholar]
- The role of plant-derived natural antioxidants in reduction of oxidative stress. BioFactors. 2022;48(3):611-633.
- [Google Scholar]
- In vitro antioxidant and α-glucosidase inhibitory activities and comprehensive metabolite profiling of methanol extract and its fractions from Clinacanthus nutans. BMC Complementary and Alternative Medicine. 2017;17(1):1-10.
- [Google Scholar]
- Phaleria macrocarpa Boerl. (Thymelaeaceae) leaves increase SR-BI expression and reduce cholesterol levels in rats fed a high cholesterol diet. Molecules. 2015;20(3):4410-4429.
- [Google Scholar]
- Anti-obesity, antioxidant and in silico evaluation of Justicia carnea bioactive compounds as potential inhibitors of an enzyme linked with obesity: Insights from kinetics, semi-empirical quantum mechanics and molecular docking analysis. Biophysical Chemistry. 2021;274:106607
- [Google Scholar]
- Antihyperlipidemic studies of newly synthesized phenolic derivatives: in silico and in vivo approaches. Drug Design, Development and Therapy 2018:2443-2453.
- [Google Scholar]
- Total phenolic content, flavonoid content and antioxidant potential of wild vegetables from Western Nepal. Plants. 2019;8(4):1-12.
- [Google Scholar]
- Extraction of bilberry (Vaccinium myrtillus) antioxidants using supercritical/subcritical CO2 and ethanol as co-solvent. Journal of Supercritical Fluids. 2016;107:358-363.
- [Google Scholar]
- Forhidrol, a bioactive fraction of Phaleria macrocarpa (Scheff.) Boerl., increases reverse cholesterol transport pathway by down-regulation of cholesteryl ester transfer protein activity. Journal of Biological Research. 2018;91(1):6863.
- [Google Scholar]
- Christina, Y. I., Rifa’i, M., Widodo, N., Djati, M. S., 2022. Comparative study of antiproliferative activity in different plant parts of Phaleria macrocarpa and the underlying mechanism of action. Sci. World J. 2022, Article ID 3992660, doi.org/10.1155/2022/3992660.
- Peroxisome proliferator-activated receptors and their agonists in nonalcoholic fatty liver disease. J. Clin. Exp. Hepatol.. 2019;9(6):731-739.
- [Google Scholar]
- Antiobesity, hepatoprotective and anti-hyperglycemic effects of a pharmaceutical formulation containing Cecropia pachystachya Trecul in mice fed with a hypercaloric diet. Journal of Ethnopharmacology. 2021;280:114418
- [Google Scholar]
- Anti-breast cancer potential activity of Phaleria macrocarpa (Scheff.) Boerl. leaf extract through in silico studies. J. Pharm. Pharmacogn. Res.. 2021;9(6):824-845.
- [Google Scholar]
- Extraction of α-glucosidase inhibitory compounds from Phaleria macrocarpa fruit flesh using solvent, sonication, and subcritical carbon dioxide Soxhlet methods. Journal of Food Biochemistry. 2017;41(5):e12399.
- [Google Scholar]
- New insights into the kinetic and thermodynamic evaluations of lipid peroxidation. Food Chemistry. 2022;375:131659
- [Google Scholar]
- Computational data of phytoconstituents from Hibiscus rosa-sinensis on various anti-obesity targets. Data in Brief. 2019;24:103994
- [CrossRef] [Google Scholar]
- The inhibition of ethanol extract of Phaleria macrocarpa stem bark on COX-2 expression of HCT116 colorectal cancer cell line. Res. J. Pharm. Technol.. 2019;12(6):2902-2906.
- [Google Scholar]
- Rapid quality evaluation of Shenzhiling oral liquid by attenuated total reflectance-infrared spectroscopy with chemometrics. Spectrochim. Acta-Part A Mol. Biomol. Spectrosc.. 2019;223:117324
- [Google Scholar]
- In-silico elucidation of phytoconstituents against 1LPB protein and anti-dyslipidaemic activity of Psoralea corylifolia Linn leaf extract. Adv. Trad. Med. 2022:1-13.
- [Google Scholar]
- Lobstein, T., Brinsden, H., Neveux, M., 2022. World Obesity Atlas 2022.
- God's crown (Phaleria macrocarpa (Scheff.) Boerl: Thymelaceae): an introduced fruit with pharmaceutical potential in the Philippines. Philipp. J. Crop Sci.. 2018;43(3):67-72.
- [Google Scholar]
- Acute and subacute toxicity assessment of liquid CO2 extract of Phaleria macrocarpa fruits flesh in mice model. J. King Saud Univ. Sci.. 2022;34(4):101912
- [Google Scholar]
- Bridging indigenous knowledge and scientific evidence for pharmacological studies of Phaleria macrocarpa: A systematic review. Natural Products Journal. 2022;12(5):29-45.
- [Google Scholar]
- In vitro, in silico and network pharmacology mechanistic approach to investigate the α-Glucosidase inhibitors identified by Q-ToF-LCMS from Phaleria macrocarpa fruit subcritical CO2 extract. Metabolites. 2022;12(12):1267.
- [Google Scholar]
- Anti-obesity drug discovery: advances and challenges. Nature Reviews. Drug Discovery. 2022;21(3):201-223.
- [Google Scholar]
- Active site-specific quantum tunneling of hACE2 receptor to assess its complexing poses with selective bioactive compounds in co-suppressing SARS-CoV-2 influx and subsequent cardiac injury. J. Adv. Vet. Anim. Res.. 2021;8(4):540.
- [Google Scholar]
- Investigation of α-glucosidase inhibitory metabolites from Tetracera scandens leaves by GC–MS metabolite profiling and docking studies. Biomolecules. 2020;10(2):287.
- [Google Scholar]
- Antiobesity effect of ethanolic extract of Ramulus mori in differentiated 3T3-L1 adipocytes and high-fat diet-induced obese mice. Journal of Ethnopharmacology. 2020;251:112542
- [Google Scholar]
- Pubchem-SID (2022): https://pubchem.ncbi.nlm.nih.gov/bioassay/1634547#sid=174489592. (Accession date 3rd August 2022).
- Oleic acid and hydroxytyrosol inhibit cholesterol and fatty acid synthesis in C6 glioma cells. Oxid. Med. Cell. Longev.. 2017;2017:9076052
- [CrossRef] [Google Scholar]
- Different extraction methods for phenolic and volatile compounds recovery from Elsholtzia ciliata fresh and dried herbal materials. Industrial Crops and Products. 2018;120:286-294.
- [Google Scholar]
- Molecular docking studies of isolated compounds from Cassia fistula on HMG-COA reductase. Asian J. Res. Chem. Pharm. Sci.. 2019;12(2):89-93.
- [Google Scholar]
- Inflammation in obesity, diabetes, and related disorders. Immunity. 2022;55(1):31-55.
- [Google Scholar]
- Correlation between dietary intake and obesity risk factors among healthy adults. Clin. Nutr. Open Sci.. 2022;45:32-41.
- [Google Scholar]
- In vitro antidiabetic activities and GC-MS phytochemical analysis of Ximenia americana extracts. S. Afr. J. Bot.. 2017;111:202-211.
- [Google Scholar]
- Time-restricted feeding suppresses excess sucrose-induced plasma and liver lipid accumulation in rats. PloS One. 2018;13(8):1-18.
- [Google Scholar]
- Trans-11 vaccenic acid dietary supplementation induces hypolipidemic effects in JCR: LA-cp rats. J. Nutr.. 2008;138(11):2117-2122.
- [Google Scholar]